Translate this page into:
Effect of forkhead box protein P2-mediated activation of myosin light-chain kinase on the invasion and migration of endometrial cancer cells
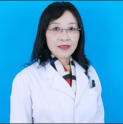
*Corresponding author: Suwen Chang, Department of Gynaecology, Yuhuangding Hospital, Yantai, Shangdong, China. csw529@126.com
-
Received: ,
Accepted: ,
How to cite this article: Chang S. The impact of forkhead box protein P2-mediated activation of myosin light-chain kinase on the invasion and migration of endometrial cancer cells. CytoJournal. 2025;22:54. doi: 10.25259/Cytojournal_31_2025
Abstract
Objective:
Endometrial cancer (EC) ranks among the most prevalent malignant tumors affecting women, with metastasis and dissemination as the major contributors to poor prognosis. This study explores the involvement of forkhead box protein P2 (FOXP2) in EC cell invasion and migration, which is mediated through the activation of myosin light-chain kinase (MYLK).
Material and Methods:
Bioinformatic analysis was conducted to determine whether FOXP2 is expressed in EC. FOXP2 overexpression was achieved using a FOXP2 overexpression vector (oeFOXP2), and negative control (NC) was used for cell transfection. Cell counting kit-8, 5-ethynyl-2’-deoxyuridine, enzyme-linked immunosorbent assay, colony formation, wound healing, and Transwell assay were used to assess the capabilities of cell viability, invasion, migration, and proliferation. Western blot and quantitative real-time polymerase chain reaction (qRTPCR) analysis were used to measure the expression levels of proteins linked to the epithelial-mesenchymal transition. The correlation between FOXP2 and MYLK was analyzed using bioinformatics and validated by Western blot and qRT-PCR analysis. The MYLK-specific inhibitor ML-7 was employed to study the impact of MYLK-mediated FOXP2 on regulating the malignant biological processes of EC.
Results:
The oeFOXP2 group of EC cells exhibited a significant decrease in cell viability, colony formation, migration rate, and metastatic cell count compared with the NC group (P < 0.05). FOXP2 overexpression markedly increased caspase-3, caspase-8, caspase-9 activity (P < 0.05). Significant changes were detected in the expression of epithelial-mesenchymal transition marker proteins, with vimentin and N-cadherin expression noticeably declining and E-cadherin expression sharply rising (P < 0.05). The addition of the MYLK-specific inhibitor ML-7 reversed the effect of FOXP2 overexpression on the invasion and migration of EC cells.
Conclusion:
FOXP2 suppresses the proliferation, invasion, and migration of EC cells through the activation of MYLK.
Keywords
Endometrial cancer
Forkhead box protein P2
Myosin light chain kinase
INTRODUCTION
Endometrial cancer (EC) is listed as one of the three most common malignant tumors in women. The incidence and fatality rates of EC have been steadily increasing in China in recent years. In 2022, 420,242 new diagnoses and 97,704 deaths due to EC were recorded.[1] Despite the generally better prognosis for EC, 13–25% of patients still experience recurrence and metastasis.[2,3] Poor prognosis is often caused by the metastasis and spread of cancer,[4] where epithelial-mesenchymal transition (EMT) is essential.[5,6] EMT plays a role in vital biological functions, such as tissue regeneration, wound healing, and embryogenesis.[7,8] Reduced E-cadherin expression in EC tissues[9,10] suggests that EMT may contribute to EC initiation and progression.
The C-terminal winged-helix/Forkhead DNA-binding domain of the FOX transcription factor family is essential for managing important biological processes, including cell division, proliferation, and overall organismal development.[11] Located on chromosome 7q31, the fox transcription factor family includes forkhead box P2 (FOXP2), which is involved in the development of the heart, lungs, and central nervous system, as well as embryonic development and cell cycle.[12-14] Furthermore, a number of cancers, including lung and breast cancer, have lower levels of FOXP2 expression.[15,16] FOXP2 may serve as a predictive biomarker and possible suppressor of metastases in breast cancer. FOXP2 aids in preventing the development and spread of breast cancer cells by modifying the EMT that is started by the transforming growth factor beta (TGF-β) signaling pathway.[17] However, further investigations are needed to comprehensively elucidate the underlying molecular mechanisms by which FOXP2 regulates the progression and pathogenesis of EC, providing deeper insights into its potential role in tumor development and therapeutic targeting.
An important part of the actin cytoskeleton is myosin light-chain kinase (MYLK).[18,19] MYLK is vital for managing numerous biological functions, including cell adhesion, migration, proliferation, and epithelial barrier development. This is achieved through its influence on the structure and reorganization of the cytoskeleton, which is essential for maintaining cellular integrity and function.[20-22] Aberrant MYLK expression alters tumor cell migration and invasion abilities, contributing to the malignant transformation of normal cells.[23-25] This study explores the involvement of FOXP2 in EC cell invasion and migration, mediated through the activation of MYLK. The ultimate goal is to identify potential molecular targets that could be used for therapeutic strategies in EC.
MATERIAL AND METHODS
Cell culture
The EC cell lines HEC-1-A (CL-0099), HEC-1-B (CL-0100), Ishikawa (CL-0283), and Human Endometrial Stromal Cells were obtained from Wuhan Pricella Biotechnology Co., Ltd. (Wuhan, China). The cells underwent STR profiling and mycoplasma testing, both of which were confirmed to be accurate. The cells were grown in Dulbecco’s modified Eagle medium/F12 medium (A4192001, Gibco BRL, Grand Island, NY, USA) added with 10% fetal bovine serum (A5670701, Gibco BRL, Grand Island, NY, USA) and 1% penicillin-streptomycin (V900929, Sigma–Aldrich, St. Louis, MO, USA). The cells were maintained at 37°C in an environment with 5% carbon dioxide (CO2) until they reached 90% confluence, at which time they were passaged using trypsin (25200056, Thermo Fisher Scientific, Waltham, MA, USA).
Cell grouping and intervention
A pcDNA3.1 expression vector with full-length FOXP2 (overexpression [oe]-FOXP2) and its negative control (NC) was produced by Shanghai GenePharma Co., Ltd. (Shanghai, China) manufactured. The transfection of cells was carried out with the Lipofectamine™ 3000 reagent (18324010, Invitrogen, Carlsbad, CA, USA).
Cells in the oeFOXP2 group were treated with the 40 μM MYLK-specific inhibitor ML-7 (HY-119038, MedChemExpress) for 24 h.[26]
Bioinformatic analysis
The “Gene_DE” module within the TIMER2.0 database (timer.cistrome.org) was applied to analyze differences in FOXP2 expression across different types of cancer. TIMER2.0 makes it easier to compare different forms of cancer with healthy control tissues using TCGA gene expression data.
FOXP2 expression was examined using the “Expression DIY” module found on the GEPIA2 website (http://gepia2.cancer-pku.cn/). The cancer type “Uterine Corpus Endometrial Carcinoma (UCEC)” was chosen, and the levels of FOXP2 in the tumor tissues of EC patients and normal uterine tissues were compared to determine any differential expression.
The Human Protein Atlas platform provided immunohistochemical pictures of FOXP2 in tumor and healthy uterine tissues (https://www.proteinatlas.org/).
The UCEC (TCGA, PanCancer Atlas) dataset was chosen after searching the “EC” database in the cBioPortal for Cancer Genomics (https://www.cbioportal.org/). After searching for FOXP2, genes that were highly linked with FOXP2 were found using the Co-expression section.
Cell proliferation assay
The cell counting kit-8 (CCK-8, C0038, Beyotime) was used to assess cell proliferation. EC cells (1 × 104 cells/well in 100 μL culture medium) were seeded in 96-well plates and incubated overnight at 37°C. Subsequently, 10 μL of CCK-8 reagent (C0038, Beyotime, Shanghai, China) was added to each well, and the cells were incubated for a further 4 h. A microplate reader (1681130, Bio-Rad Laboratories, Hercules, USA) was employed to measure absorbance at 450 nm.
Cells from each group were reseeded in 96-well plates at a density of 1 × 104 cells/well, and after 48 h of incubation, 5-Ethynyl-2’-deoxyuridine (EdU) was added to each well and incubated for a further 2 h and 4’,6-Diamidino-2’-phenylindole (BS097, Biosharp Life Science, Hefei, Anhui, China) for 15 min. Fluorescence pictures were taken using a fluorescence microscope (DM3000, Leica, Wetzlar, Germany), and the EdU-555 Cell Proliferation Detection Kit (C0075L, Beyotime, Shanghai, China) procedure was followed. EdU-positive and total cells were measured using ImageJ software (v1.48, NIH, Bethesda, MD, USA). Cell proliferation rate was computed as follows: Proliferation rate = (EdU-positive cells/Total cells) × 100%.
Cysteinyl aspartate specific proteinase (caspase) activity
Apoptosis was assessed using the caspase-3 activity assay kit (C1115, Beyotime, Shanghai, China), caspase-8 activity assay kit (C1151, Beyotime, Shanghai, China), and caspase-9 activity assay kit (C1157, Beyotime, Shanghai, China). Briefly, cells were harvested and lysed with radio-immunoprecipitation assay buffer. The reagents were added based on the instructions from the manufacturer, and the activity was assessed by measuring absorbance at 450 nm.
Colony formation assay
EC cells were grown at 37°C with 5% CO2 following their seeding into 6-well plates at a density of 200 cells/well. After around 10 days, colony development was noticed, and the medium was replaced every 3 days. 0.5% crystal violet (C0121, Beyotime, Shanghai, China) was used to stain the colonies, and 4% paraformaldehyde (P0099, Beyotime, Shanghai, China) was used to fix them. ImageJ software (version 1.48, NIH, Bethesda, MD, USA) was used to quantify the number of colonies.
Wound healing assay
On the bottom of a 24-well plate, six parallel lines were marked. EC cells were placed onto the plate following trypsin digestion. After the cells achieved 90% confluence, the creation of linear scratches involved a 10 μL pipette tip. Serum-free medium was reintroduced into the wells and incubated for a further 24 h after any leftover cellular debris had been removed with three PBS washes. The wound sizes were measured at 0, 12, and 24 h with the help of an inverted microscope (DMi8, Zeiss, Oberkochen, Baden-Württemberg, Germany).
Transwell assay
The top compartment of the Transwell plate (355467, Corning, NY, USA) was filled with 200 μL of Matrigel that had been diluted with serum-free media in the proper ratio to measure cell movement. A sterile laminar flow hood was used to allow the Matrigel gel (354234, Corning, NY, USA) to dry. For the migration test, treated cells (2 × 104 cells/well) were planted into the top compartment, while the lower chamber contained complete media and the top chamber contained serum-free medium. After 48 h, 1% crystal violet (C0121, Beyotime, Shanghai, China) was used to stain the migratory cells after they had been fixed with 4% paraformaldehyde. Using a microscope (DMi8, Zeiss, Oberkochen, Baden-Württemberg, Germany), pictures of the labeled cells were captured.
Western blot
Proteins were measured using the Bicinchoninic Acid Protein Assay Kit (P0012, Beyotime). After the samples were separated using 10% sodium dodecyl sulfate-polyacrylamide gel electrophoresis, they were put onto polyvinylidene fluoride membranes (IPVH00010, Merck Millipore, Billerica, MA, USA). A 5% (v/v) non-fat milk solution was used to block the main antibody, which was then incubated at 4°C for the entire night. After being washed, the membrane underwent a 1-h treatment at room temperature with a secondary antibody and horseradish peroxidase (HRP). Enhanced chemiluminescence (34577, Thermo Fisher Scientific, Waltham, MA, USA) was used to identify protein signals, and the ChemiDoc Imaging System (Bio-Rad, Hercules, CA, USA) was used for analysis. The primary antibodies utilized were glyceraldehyde-3-phosphate dehydrogenase (GAPDH, ab8245), anti-FOXP2 (1:1000, ab1307), anti-E-cadherin (1:1000, ab238099), anti-Vimentin (1:1000, ab92547), and anti-N-cadherin (1:1000, ab245117), and anti-MYLK (1:1000, ab1307). The secondary antibodies were goat anti-mouse immunoglobulin G [IgG] H&L (HRP) (ab205719, 1:10000) and goat anti-rabbit IgG H&L (ab205718, 1:10000). We bought all of the antibodies from Abcam (Cambridge, MA, USA).
Quantitative reverse-transcription polymerase chain reaction (qRT-PCR)
Total RNA was extracted with the help of TRIzol reagent (T9424, Sigma-Aldrich, St. Louis, MO, USA), with subsequent incubation at 65°C for 5 min and 4°C for 2 min. The process of reverse transcription was executed with the PrimeScript RT Reagent Kit (RR037A, TaKaRa, Tokyo, Japan). qPCR was conducted on a LightCycler 96 thermocycler (Roche, Shanghai, China) using the SYBR Premix ExTaq Kit (639503, TaKaRa, Tokyo, Japan) following the guidelines provided by the manufacturer. The sequences of primers can be found in Table 1. Gene expression was evaluated using the 2−△△CT method, with GAPDH as the internal control and a control group sample as the reference.
Gene | Accession number | Primer sequences |
---|---|---|
FOXP2 | NM_148898 | F: 5'-TGGATGACCGAAGCACTGCTCA-3' R: 5'-TGGGAGATGGTTTGGGCTCTGA-3' |
E-cadherin | NM_004360 | F: 5'-GCCTCCTGAAAAGAGAGTGGAAG-3' R: 5'-TGGCAGTGTCTCTCCAAATCCG-3' |
Vimentin | NM_003380 | F: 5'-AGGCAAAGCAGGAGTCCACTGA-3' R: 5'-ATCTGGCGTTCCAGGGACTCAT-3' |
N-cadherin | NM_001792 | F: 5'-CCTCCAGAGTTTACTGCCATGAC-3' R: 5'-GTAGGATCTCCGCCACTGATTC-3' |
GAPDH | NM_002046 | F: 5'-GTCTCCTCTGACTTCAACAGCG-3' R: 5'-ACCACCCTGTTGCTGTAGCCAA-3' |
FOXP2: Forkhead box protein P2, GAPDH: Glyceraldehyde-3-phosphate dehydrogenase, A: Adenine, C: Cytosine, G: Guanine, T: Thymine
Statistical analysis
The data were analyzed using GraphPad (GraphPad Software, San Diego, CA, USA) and tested for normality using the Shapiro–Wilk test; normally distributed continuous variables are displayed as mean ± standard deviation (x̄± s); multiple groups were compared using analysis of variance, and pairwise comparisons were made using the least significant difference-t-test; and a P-value of under 0.05 indicated statistical significance.
RESULTS
FOXP2 is poorly expressed in EC cells
FOXP2 expression in EC and normal tissues was compared using the TIMER2.0 and GEPIA2 databases. Differential expression of FOXP2 across various malignant tumors was identified through analysis using the TIMER2.0 database [Figure 1a]. In EC tissues, FOXP2 expression was considerably reduced compared to that in endometrial tissues, according to analysis from the GEPIA2 database, which suggests that it may have a suppressor function in the development of cancer [Figure 1b]. Furthermore, immunohistochemical data from the Human Protein Atlas database demonstrated a marked reduction in FOXP2 protein expression in EC tissues, further reinforcing its potential clinical significance in this cancer type [Figure 1c and d].
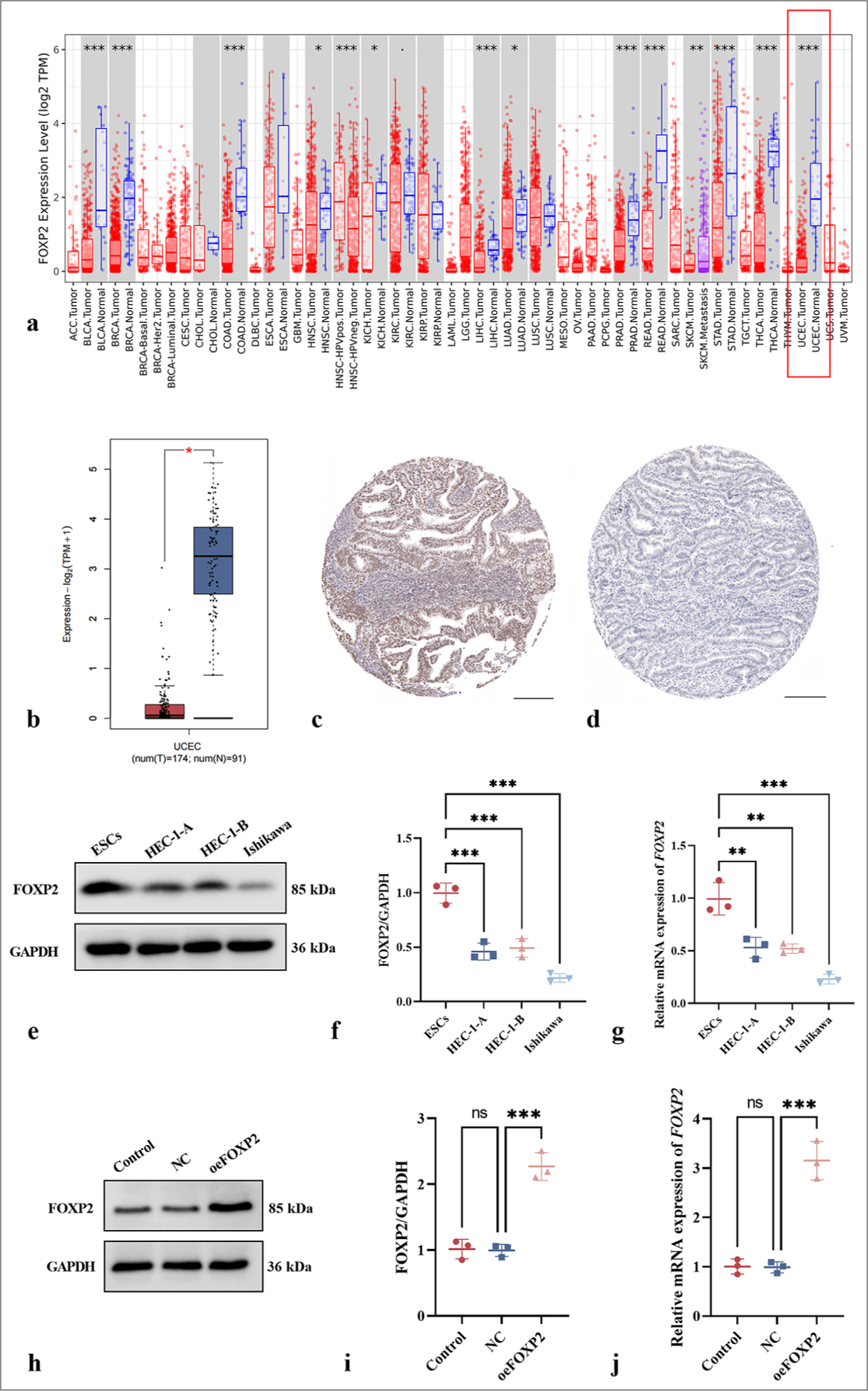
- Differential expression of FOXP2 in EC. (a) Differential expression of FOXP2 across various malignant tumors (TIMER2.0 Database). (b) Comparison of FOXP2 expression in EC and normal tissues (GEPIA2 Database). (c) Immunohistochemical analysis of FOXP2 expression in EC tissues (human protein atlas). (d) Immunohistochemical analysis of FOXP2 expression in normal tissues (human protein atlas). (e-g) FOXP2 expression in normal endometrial stromal cells and EC cell lines. (h-j) Transfection efficiency assessment in Ishikawa cells (FOXP2 overexpression model). Scale bars: 100 μm. The experiments were carried out in sets of three, with the outcomes reported as the mean (n = 3). ns: No significance. ✶P < 0.05, ✶✶P < 0.01, ✶✶✶P < 0.001. FOXP2: Forkhead box protein P2, NC: Negative control, oeFOXP2: Overexpression FOXP2, GAPDH: Glyceraldehyde-3-phosphate dehydrogenase, ESCs: Human endometrial stromal cells, ACC: Adrenocortical carcinoma, BLCA: Bladder urothelial carcinoma, BRCA: Breast invasive carcinoma, CESC: Cervical squamous cell carcinoma and endocervical adenocarcinoma, CHOL: Cholangiocarcinoma, COAD: Colon adenocarcinoma, DLBC: Diffuse large B-cell lymphoma, ESCA: Esophageal carcinoma, GBM: Glioblastoma multiforme, HNSC: Head and neck squamous cell carcinoma, KICH: Kidney chromophobe, KIRC: Kidney renal clear cell carcinoma, KIRP: Kidney renal papillary cell carcinoma, LAML: Acute myeloid leukemia, LGG: Lower-grade glioma, LIHC: Liver hepatocellular carcinoma, LUAD: Lung adenocarcinoma, LUSC: Lung squamous cell carcinoma, MESO: Mesothelioma, OV: Ovarian serous cystadenocarcinoma, PAAD: Pancreatic adenocarcinoma, PCPG: Pheochromocytoma and paraganglioma, PRAD: Prostate adenocarcinoma, READ: Rectum adenocarcinoma, SARC: Sarcoma, SKCM: Skin cutaneous melanoma, STAD: Stomach adenocarcinoma, TGCT: Testicular germ cell tumors, THCA: Thyroid carcinoma, THYM: Thymoma, UCEC: Uterine corpus endometrial carcinoma, UCS: Uterine carcinosarcoma, UVM: Uveal melanoma.
The bioinformatics analysis findings were further validated at the cellular level using Western blot and qRT-PCR experiments. The analysis indicated that FOXP2 expression levels were significantly higher in normal endometrial stromal cells than in the EC cell lines HEC-1-A, HEC-1-B, and Ishikawa [Figure 1e-g]. This result aligns with the data from the TIMER2.0 and GEPIA2 databases, providing additional support for the potential tumor-suppressive role of FOXP2. Ishikawa cells were selected for additional study because they showed the lowest expression of all of the cell lines (P < 0.05).
A FOXP2 overexpression model was established in the Ishikawa EC cell line to examine how FOXP2 affects EC cells’ biological processes. Transfection efficiency was assessed through immunoblotting and qRT-PCR, which demonstrated that 24 h post-transfection, the oeFOXP2 group showed a marked increase in FOXP2 expression (P < 0.05), whereas the NC groups showed no discernible differences (P > 0.05) [Figure 1h-j]. This finding indicates that transfection was both specific and effective.
FOXP2 suppresses the viability and proliferation of EC cells
FOXP2 overexpression suppresses DNA synthesis and cell proliferation, as indicated by the CCK-8 assay, which showed significantly lower cell proliferation in the oeFOXP2 group than in NC group (P < 0.05) [Figure 2a]; the colony formation assay, which showed fewer colonies in the oeFOXP2 group, indicating impaired long-term proliferative capacity (P < 0.05) [Figure 2b and c]; and a significant decline in EdU-positive cells was observed in the oeFOXP2 group according to the EdU assay (P < 0.05) [Figure 2d and e]. FOXP2 overexpression markedly increased caspase-3, caspase-8, caspase-9 activity (P < 0.05) [Figure 2f-h].
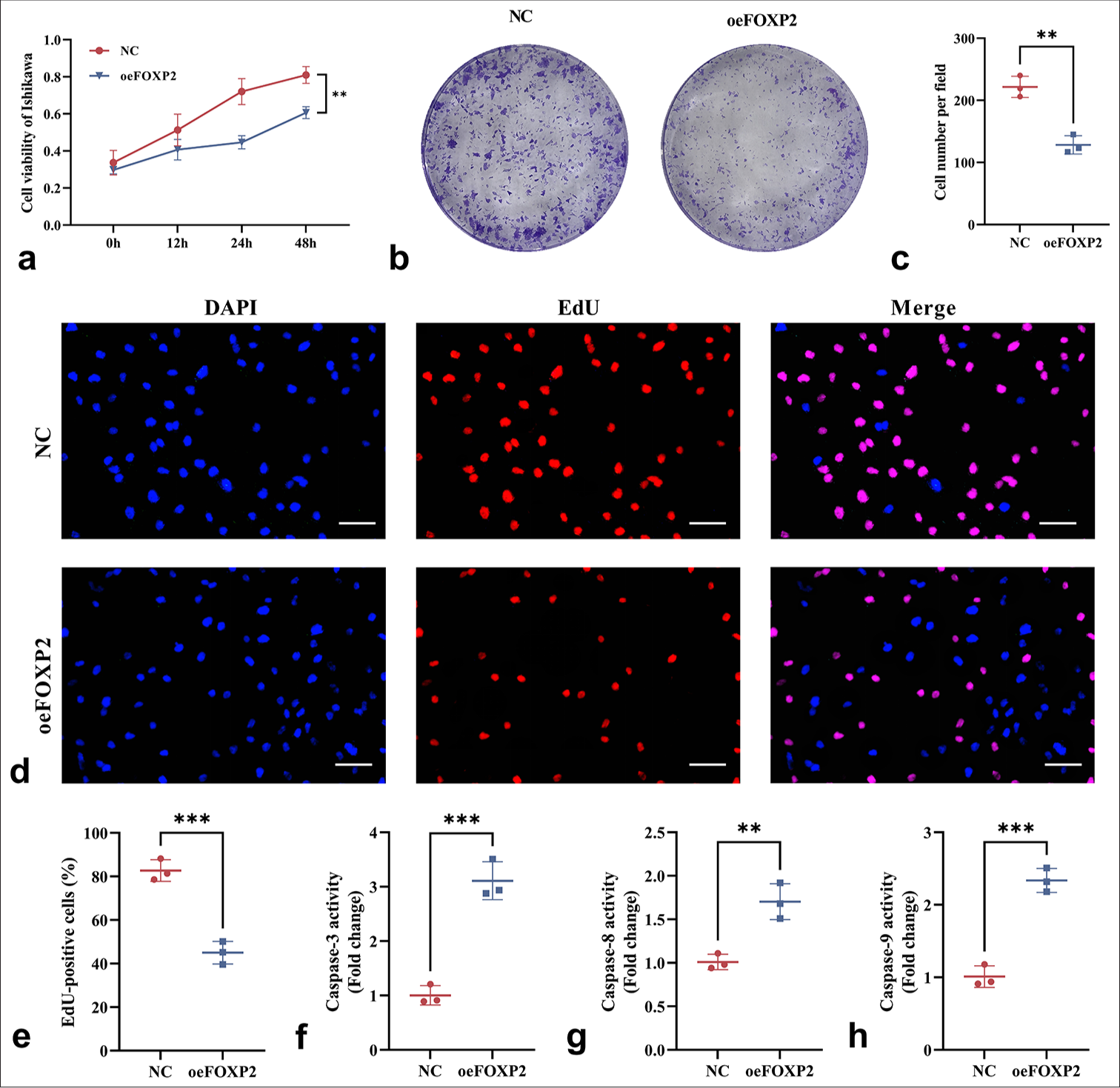
- Impact of FOXP2 overexpression on the viability and proliferation of EC cells. (a) CCK-8 assay showing reduced cell viability in the oeFOXP2 Group. (b and c) Colony formation assay revealing a decrease in colony number in the oeFOXP2 Group. (d and e) EdU assay showing a reduction in the proportion of EdU-positive cells in the oeFOXP2 Group. (f-h) ELISA kits were used to evaluate the activities of caspase-3, caspase-8, and caspase-9. Scale bars: 25 μm (×400 magnification). The experiments were carried out in sets of three, with the outcomes reported as the mean (n = 3). ✶✶P < 0.01, ✶✶✶P < 0.001. EC: Endometrial cancer, CCK-8: Cell counting kit-8, FOXP2: Forkhead box protein P2, NC: Negative control, oeFOXP2: Overexpression FOXP2, EdU: 5-Ethynyl-2’-deoxyuridine, DAPI: 4’,6-Diamidino-2’-phenylindole, caspase: Cysteinyl aspartate specific proteinase, ELISA: Enzyme-linked immunosorbent assay.
FOXP2 limits the ability of EC cells to migrate and invade
The migratory distance of cells in the oeFOXP2 group was substantially less than in the NC group (P < 0.05), according to the wound healing experiment [Figure 3a and b]. This implies that the overexpression of FOXP2 effectively prevented the EC cells from migrating. FOXP2 inhibits EC cell invasion, as demonstrated by the transwell experiment, which showed that the oeFOXP2 group had a considerably lower cell count and decreased invasion capacity than in NC group (P < 0.05) [Figure 3c and d].
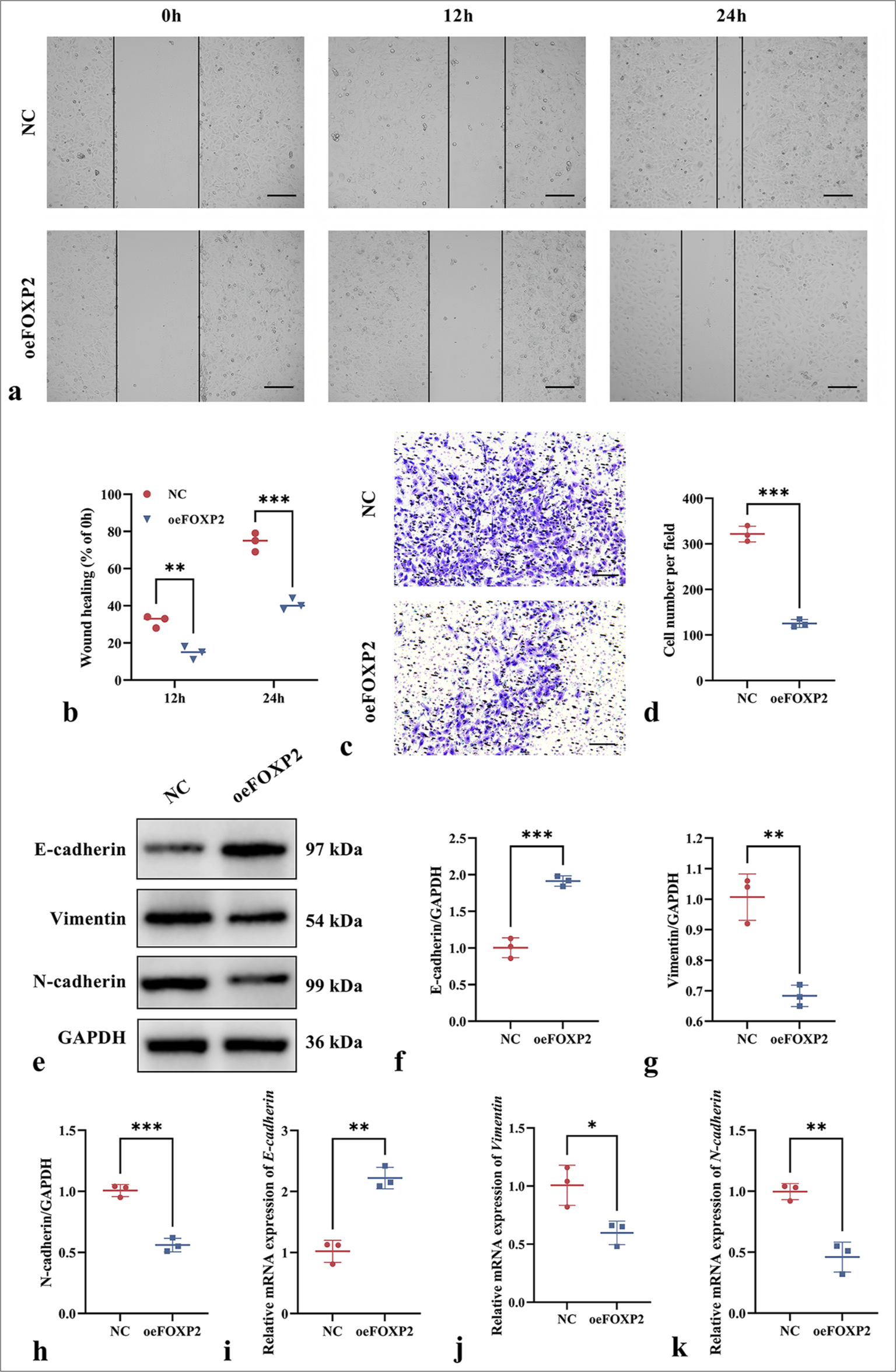
- Impact of FOXP2 overexpression on migration and invasion on EC cells. (a and b) wound healing assay showing reduced migration in the oeFOXP2 group. (c and d) Transwell assay demonstrating decreased invasion of cells in the oeFOXP2 group. (e-k) EMT marker expression in the oeFOXP2 group. Scale bars: 50 μm (×200 magnification). The experiments were carried out in sets of three, with the outcomes reported as the mean (n = 3). ✶P < 0.05, ✶✶P < 0.01, ✶✶✶P < 0.001. EC: Endometrial cancer, FOXP2: Forkhead box protein P2, NC: Negative control, oeFOXP2: Overexpression FOXP2, GAPDH: Glyceraldehyde-3-phosphate dehydrogenase.
The oeFOXP2 group showed significant changes in EMT marker expression, as determined by Western blot and qRT-PCR analysis. In particular, vimentin and N-cadherin expression dropped while E-cadherin expression rose (P < 0.05) [Figure 3e-k], indicating that FOXP2 inhibits the EMT process to reduce cell migration and invasion. The findings imply that FOXP2 may control the EMT process to prevent EC cell migration and invasion, thus acting as a suppressor in the malignant transformation of EC.
FOXP2 modulates MYLK expression
A significant positive correlation between FOXP2 and MYLK (P < 0.05) was identified through bioinformatics analysis using cBioPortal [Figure 4a], indicating that FOXP2 may control MYLK expression. According to an analysis of data from the GEPIA2 database, MYLK expression was considerably lower in EC tissues (P < 0.05) than in normal tissues [Figure 4b], indicating that MYLK may be a focus for more research. As such, we continued to investigate whether FOXP2 regulates the MYLK pathway.
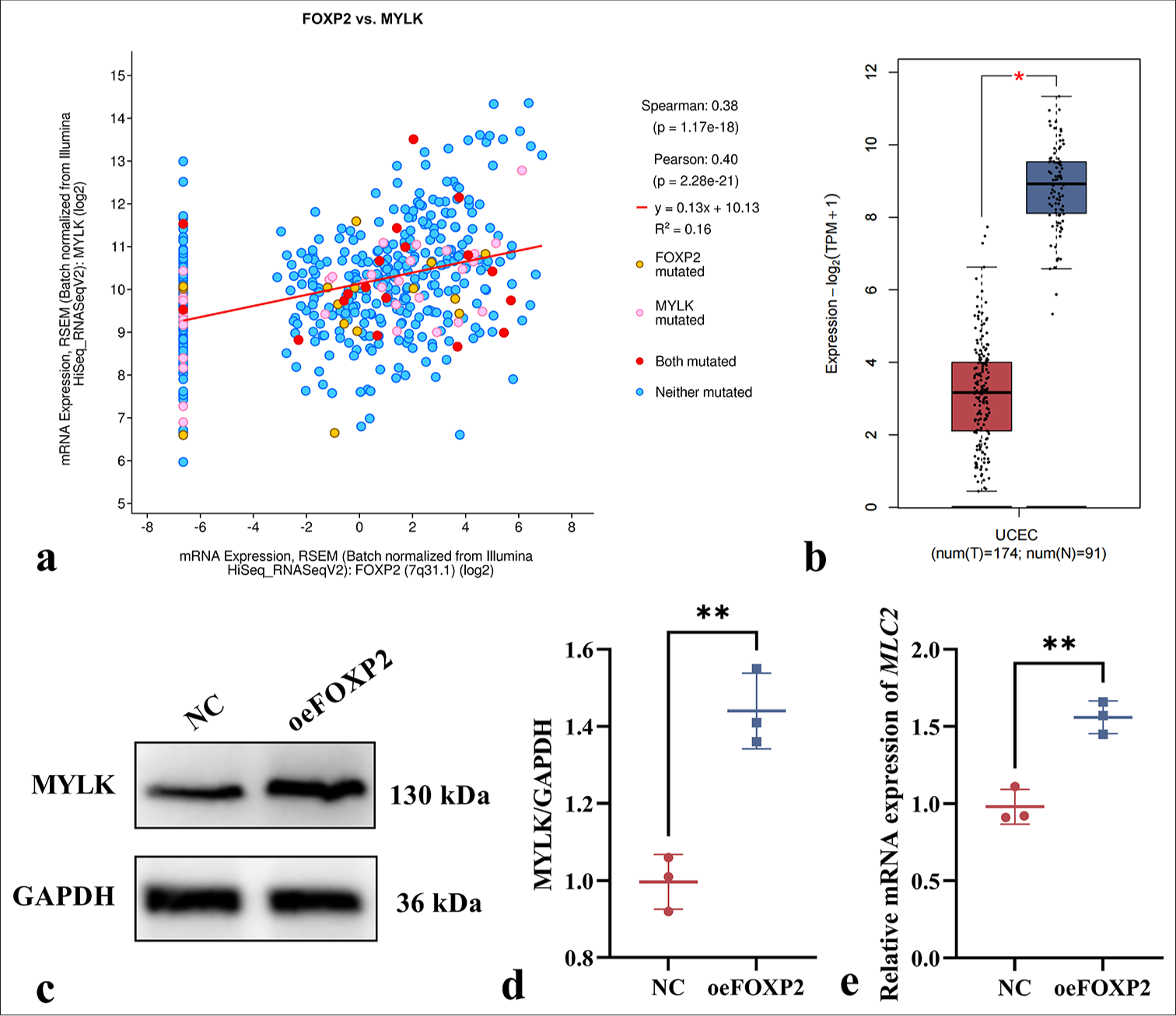
- FOXP2 regulates MYLK expression in EC cells. (a) Bioinformatics analysis revealing a significant positive correlation between FOXP2 and MYLK expression (cBioPortal). (b) MYLK expression is significantly decreased in EC tissues compared to normal tissues (GEPIA2 Database). (c-e) The expression levels of MYLK. The experiments were carried out in sets of three, with the outcomes reported as the mean (n = 3). ✶P < 0.05, ✶✶P < 0.01. EC: Endometrial cancer, FOXP2: Forkhead box protein P2, MYLK: Myosin light chain kinase, NC: Negative control, oeFOXP2: overexpression FOXP2, GAPDH: Glyceraldehyde-3-phosphate dehydrogenase.
Western blot and qRT-PCR analysis were carried out on the NC and oeFOXP2 groups in this investigation to assess the expression levels of MYLK at both the protein and mRNA levels. The findings showed that MYLK had considerably higher protein levels in the oeFOXP2 group (P < 0.05) [Figure 4c-e]. This further supports the regulatory role of FOXP2 in the MYLK signaling pathway by indicating that increasing FOXP2 levels can enhance the expression of both MYLK. These results imply that FOXP2 is positively correlated with the modulation of MYLK expression.
MYLK mediates the inhibitory effect of FOXP2 on the malignant biological processes of EC
This work investigated the role of MYLK in the regulatory pathways by which FOXP2 affects the malignant biological processes in EC. To gain insights into possible molecular targets for treatment approaches, we sought to understand how the connection between FOXP2 and MYLK contributes to the aggressiveness and development of EC. By treating oeFOXP2 cells with the MYLK-specific inhibitor ML-7, we carried out a number of tests to assess whether MYLK inhibition affected cell invasion, migration, proliferation, and EMT.
First, the CCK-8 experiment revealed that the MYLK inhibitor ML-7 administration markedly increased the proliferation of oeFOXP2 cells (P < 0.05) [Figure 5a], underscoring the critical function of MYLK in EC cell proliferation. This conclusion was corroborated by the colony formation experiment, which showed that ML-7-treated oeFOXP2 cells produced a statistically significant (P < 0.05) increase in the number of colonies when compared with those untreated group [Figure 5b and c]. In addition, a significantly larger proportion of EdU-positive cells was observed in the ML-7 treatment group (P < 0.05), indicating that the EdU test validated the boosting impact of MYLK activation on DNA synthesis [Figure 5d and e]. Furthermore, caspase activity assays revealed that ML-7 treatment led to a significant reduction in caspase-3, caspase-8, and caspase-9 activity (P < 0.05), suggesting that MYLK inhibition mitigates apoptosis in oeFOXP2 cells, thereby promoting cell survival [Figure 5 f-h].
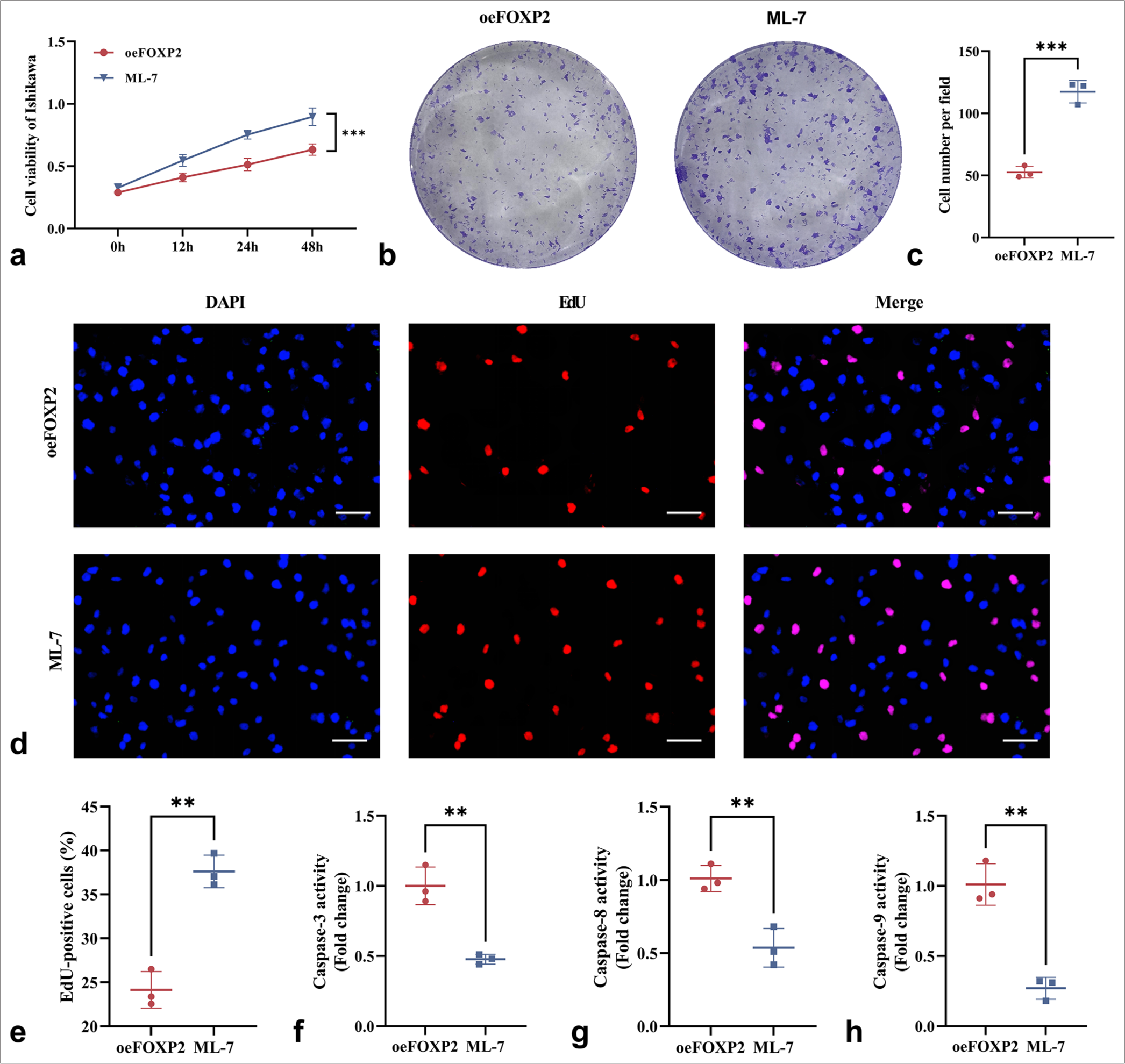
- ML-7 enhances the cell activity of EC cells. (a) CCK-8 assay showing increased proliferation of oeFOXP2 cells following ML-7 treatment. (b and c) Colony formation assay demonstrating a higher number of colonies in the ML-7-treated group. (d and e) EdU assay revealing an increased proportion of EdU-positive cells upon ML-7 treatment. (f-h) The activities of caspase-3, caspase-8, and caspase-9. Scale bars: 25 μm (×400 magnification). The experiments were carried out in sets of three, with the outcomes reported as the mean (n = 3). ✶✶P < 0.01, ✶✶✶P < 0.001. EC: Endometrial cancer, FOXP2: Forkhead box protein P2, ML-7: MYLK-specific inhibitor, oeFOXP2: Overexpression FOXP2, EdU: 5-Ethynyl-2’-deoxyuridine, DAPI: 4’,6-Diamidino-2’-phenylindole, caspase: Cysteinyl aspartate specific proteinase, CCK-8: Cell counting kit-8.
MYLK inhibitor ML-7 inhibition significantly enhanced the migration and invasion of oeFOXP2 cells, highlighting that MYLK plays a key role in promoting EC cell migration and invasion (P < 0.05) [Figure 6a-d]. The downregulation of E-cadherin (P < 0.05) and the higher expression of N-cadherin and Vimentin (P < 0.05) were further indications from Western blot and qRT-PCR analysis of EMT-related genes that MYLK inhibitor ML-7 significantly enhanced EMT marker alterations [Figure 6e-k]. These results imply that MYLK inhibitor ML-7 may support the EMT process, which may contribute to the malignant biological activity of EC cells.
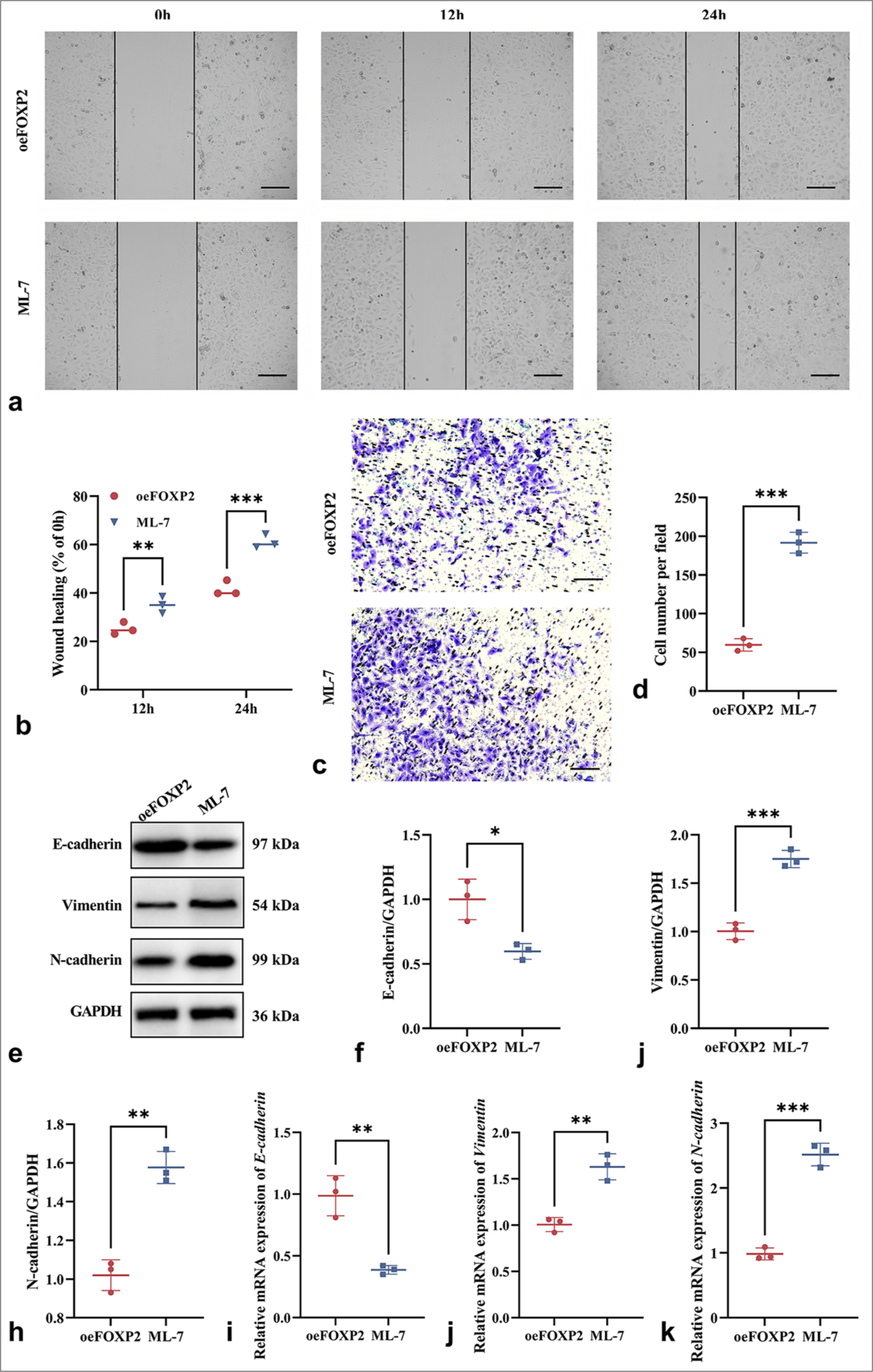
- ML-7 enhances the malignant phenotypes of EC cells. (a and b) Wound healing assay showing enhanced migration ability in ML-7-treated oeFOXP2 cells. (c and d) Transwell assay indicating increased migration and invasion capabilities following ML-7 treatment. (e-k) EMT Marker Expression in ML-7-treated oeFOXP2 Group. Scale bars: 50 μm (×200 magnification). The experiments were carried out in sets of three, with the outcomes reported as the mean (n = 3). ✶P < 0.05, ✶✶P < 0.01, ✶✶✶P < 0.001. EC: Endometrial cancer, EMT: Epithelial– mesenchymal transition, FOXP2: Forkhead box protein P2, ML-7: MYLK-specific inhibitor, oeFOXP2: Overexpression FOXP2, GAPDH: Glyceraldehyde-3-phosphate dehydrogenase.
When combined, MYLK is a key biological player in mediating the acceleration of EC cell migration, invasion, proliferation, and EMT that is caused by FOXP2.
DISCUSSION
With a rising incidence rate globally, EC is among the three primary malignant tumors affecting the female reproductive system. Its underlying pathophysiology is yet unknown, though. Prolonged exposure to elevated estrogen levels is widely recognized as a significant risk factor contributing to the initiation and progression of EC, as it disrupts hormonal homeostasis and promotes aberrant cellular proliferation within the endometrial tissue. Furthermore, the onset and progression of the disease are largely influenced by the dysregulation of key genetic pathways, including the turning off of genes that act as tumor suppressors and the aberrant activation of oncogenes.[27,28] Advancements in clinical diagnostic techniques have led to an increased detection rate of EC. The condition poses a significant threat to reproductive health in women, which is still linked to a high risk of metastasis and recurrence.[29,30]
A growing body of research has extensively documented the pivotal role of FOXP2 in the development and progression of multiple cancer types. In particular, it is discovered that FOXP2 expression is markedly downregulated in thyroid cancer tissues and cell lines. Promoting cell division and preventing apoptosis, this downregulation of FOXP2 aids in the development of thyroid cancer. In addition, it has been demonstrated that FOXP2 controls important signaling pathways, including the transcription of ribosomal protein S6 kinase α6, which is essential for regulating cell growth and survival. This points to the fact that FOXP2 is believed to play a pivotal role in regulating key cellular processes associated with carcinogenesis and may act as a tumor suppressor in thyroid cancer by modulating pathways involved in proliferation, invasion, and metastatic progression.[31] In breast cancer, FOXP2 could be a new prognostic indicator and metastasis suppressor. FOXP2 plays a vital role in curbing the proliferation, invasion, and metastasis of breast cancer cells by modulating the EMT process initiated by the TGF-β signaling pathway, thereby hindering tumor progression.[17] In individuals with colorectal cancer, low expression of FOXP2 has been closely linked to worse survival results. Numerous investigations have revealed a notable downregulation of FOXP2 in the tissues of colorectal cancer, indicating that it may have a tumor suppressor function. Colorectal cancer cells become more invasive when FOXP2 is downregulated.[32] In addition, using RNA immunoprecipitation sequencing, scientists have shown that the long non-coding RNA (lncRNA) SNHG5 is an upstream gene that directly controls the FOXP2 protein. In a uterine adhesion cell model, the downregulation of lncRNA SNHG5 inhibited the expression of FOXP2 protein, thereby altering the extracellular matrix accumulation in vitro in endometrial stromal cells.[33] Using bioinformatic techniques, this research began by analyzing the expression levels of FOXP2 in EC. The findings demonstrated a noteworthy reduction in FOXP2 protein expression in EC tissues, corroborating its possible therapeutic relevance in the setting of EC. Consistent with previous studies, this research demonstrates that FOXP2 overexpression inhibits the proliferation and metastasis of EC cells, implying an anti-tumor role for FOXP2 in the progression of EC. By controlling the EMT process, FOXP2 may prevent EC cells from migrating and invading, preventing the EC cells from becoming cancerous.
The expression of MYLK and FOXP2 in this study showed a strong positive association, according to bioinformatics analysis conducted with cBioPortal, suggesting that FOXP2 may regulate the expression of MYLK. MYLK phosphorylates at Ser19 to control MYL2.[34] MYL2 phosphorylation alters the actin cytoskeleton by encouraging cell contraction and motility.[35] The cytoskeleton must change swiftly and dynamically for cancer cells to penetrate and spread.[36] Previous studies have demonstrated that MYLK is a key regulator of cell migration across multiple malignancies, including breast, prostate, gastric, liver, lung, and bladder cancers, as well as melanoma. Its dysregulation has been implicated in tumor progression, metastasis, and the acquisition of invasive phenotypes.[36,37] The activation of MYLK has been demonstrated to influence the actin cytoskeleton and cell adhesion, thereby enhancing the migratory ability of tumor cells. Therefore, the pivotal function of MYLK in the progression of different cancers suggests that it could be a key therapeutic target for controlling tumor invasion and metastasis. Its involvement in key oncogenic pathways makes it a promising candidate for future research. These studies have identified MYLK as a key player in promoting the motility and invasiveness of cancer cells, which are critical factors in cancer metastasis. There are several uses for selective MYLK inhibition using ML-7, such as delaying tumor development and making MYLK-expressing cancer cells more susceptible to apoptosis.[38,39] By introducing the MYLK-specific inhibitor ML-7 into oeFOXP2 cells, we evaluated the effects of MYLK inhibition on the migration, invasion, proliferation, and EMT of EC cells. The findings suggest that MYLK is essential in mediating the regulatory effects of FOXP2 on the EMT process. The suppression of FOXP2 overexpression on the malignant behaviors of EC cells, such as their proliferative, migratory, and invasive capacities, was dramatically reversed when MYLK was inhibited. This suggests that MYLK is a crucial downstream effector in the way that FOXP2 regulates the EMT process and that inhibiting MYLK may help EC cells regain or improve their aggressive characteristics. These results offer an understanding of the molecular processes in cancer progression by focusing on how FOXP2 and MYLK interactions influence the malignant activities of EC cells.
The findings of the research imply that FOXP2 may trigger the MYLK signaling pathway to prevent EC cell migration and proliferation in vitro. While our research offers fresh perspectives on the function of FOXP2 in EC and its interaction with MYLK, several limitations should be considered. First, our findings are based on in vitro experiments, necessitating in vivo validation. Second, the precise mechanism of FOXP2-MYLK regulation remains unclear, requiring further mechanistic studies. Third, the assessment of FOXP2 nuclear localization in response to overexpression, which could provide insights into its mechanism of action, was not conducted in this study. Fourth, only one cell line was used in our experiments, which could restrict how broadly our findings can be applied. Using multiple cell lines, one could gain a more complete understanding of how FOXP2 functions in EC. Finally, its involvement in other oncogenic pathways beyond EMT regulation should be examined. Addressing these limitations will enhance our understanding of FOXP2 in EC and support the development of targeted therapies.
SUMMARY
Our research shows that FOXP2 is essential for controlling the biological activities of EC cells that are malignant. Through the activation of the MYLK signaling pathway, it has been discovered that FOXP2 overexpression significantly suppresses cell invasion, migration, and proliferation, and FOXP2 is a promising target for EC treatment.
ACKNOWLEDGMENT
Not applicable.
AVAILABILITY OF DATA AND MATERIALS
The data that support the findings of this study are available from the corresponding author upon reasonable request.
ABBREVIATIONS
BCA: Bicinchoninic Acid
caspase: Cysteinyl aspartate specific proteinase
CCK-8: Cell Counting Kit-8
DAPI: 4’,6-Diamidino-2’-phenylindole
DMEM: Dulbecco’s Modified Eagle Medium
EC: Endometrial cancer
EdU: 5-Ethynyl-2’-deoxyuridine
ELISA: Enzyme-linked immunosorbent assay
EMT: Epithelial-mesenchymal transition
ESCs: Endometrial Stromal Cells
FOXP2: Forkhead box protein P2
GAPDH: Glyceraldehyde-3-phosphate dehydrogenase
HRP: Horseradish peroxidase
MYLK: Myosin light chain kinase
NC: Negative control
oeFOXP2: Overexpression FOXP2
PVDF: Polyvinylidene fluoride
qRT-PCR: Quantitative real-time polymerase chain reaction
SDS-PAGE: Sodium dodecyl sulfate-polyacrylamide gel electrophoresis
TGF-β: Transforming growth factor beta
UCEC: Uterine corpus endometrial carcinoma
AUTHOR CONTRIBUTIONS
SWC: Conceived and designed the study; SWC: Responsible for data acquisition; SWC: Analyzed and interpreted the data; SWC: Responsible for experiments; SWC: Drafted the first version of the manuscript; SWC: Critically revised the manuscript. All authors meet ICMJE authorship requirements.
ETHICS APPROVAL AND CONSENT TO PARTICIPATE
Ethical approval and consent to participate is not required as this study did not include animal experiments, and the patient data are sourced from the database.
CONFLICT OF INTEREST
The authors declare that they have no conflict of interest.
EDITORIAL/PEER REVIEW
To ensure the integrity and highest quality of CytoJournal publications, the review process of this manuscript was conducted under a double-blind model (authors are blinded for reviewers and vice versa) through an automatic online system.
FUNDING: The authors received no financial support for the research, authorship, or publication of this article.
References
- Global cancer statistics 2022: GLOBOCAN estimates of incidence and mortality worldwide for 36 cancers in 185 countries. CA Cancer J Clin. 2024;74:229-63.
- [CrossRef] [PubMed] [Google Scholar]
- ERRα Up-regulates invadopodia formation by targeting HMGCS1 to promote endometrial cancer invasion and metastasis. Int J Mol Sci. 2023;24
- [CrossRef] [PubMed] [Google Scholar]
- The molecular mechanisms and therapeutic strategies of EMT in tumor progression and metastasis. J Hematol Oncol. 2022;15:129.
- [CrossRef] [PubMed] [Google Scholar]
- EMT, MET, Plasticity, and tumor metastasis. Trends Cell Biol. 2020;30:764-76.
- [CrossRef] [PubMed] [Google Scholar]
- Molecular mechanism involved in epithelial to mesenchymal transition. Arch Biochem Biophys. 2021;710:108984.
- [CrossRef] [PubMed] [Google Scholar]
- The Epithelial-to-mesenchymal transition (EMT) in development and cancer. Annu Rev Cancer Biol. 2020;4:197-220.
- [CrossRef] [PubMed] [Google Scholar]
- The Role of ROR1 in chemoresistance and EMT in endometrial cancer cells. Medicina (Kaunas). 2023;59:994.
- [CrossRef] [PubMed] [Google Scholar]
- Netrin-1 blockade inhibits tumour growth and EMT features in endometrial cancer. Nature. 2023;620:409-16.
- [CrossRef] [PubMed] [Google Scholar]
- FOXO transcription factors as therapeutic targets in human diseases. Trends Pharmacol Sci. 2022;43:1070-84.
- [CrossRef] [PubMed] [Google Scholar]
- Lateral parabrachial FoxP2 neurons regulate respiratory responses to hypercapnia. Nat Commun. 2024;15:4475.
- [CrossRef] [PubMed] [Google Scholar]
- BMP7 expression in mammalian cortical radial glial cells increases the length of the neurogenic period. Protein Cell. 2024;15:21-35.
- [CrossRef] [PubMed] [Google Scholar]
- FOXP transcription factors in vertebrate brain development, function, and disorders. Wiley Interdiscip Rev Dev Biol. 2020;9:e375.
- [CrossRef] [PubMed] [Google Scholar]
- FOXP2 inhibits the aggressiveness of lung cancer cells by blocking TGFβ signaling. Oncol Lett. 2024;27:227.
- [CrossRef] [PubMed] [Google Scholar]
- Study on the mechanism of FOXA2 activation on glutathione metabolic reprogramming mediated by ETV4 transcription to facilitate colorectal cancer malignant progression. Biochem Genet 2024 Available from: http://dx.doi.org/10.1007/s10528-024-10918-y
- [CrossRef] [Google Scholar]
- Comprehensive analysis of prognostic and immune infiltrates for FOXPs transcription factors in human breast cancer. Sci Rep. 2022;12:8896.
- [CrossRef] [PubMed] [Google Scholar]
- Actin finally matures: Uncovering machinery and impact. Trends Biochem Sci. 2023;48:414-6.
- [CrossRef] [PubMed] [Google Scholar]
- Phosphorylation-dependent subcellular redistribution of small myosin light chain kinase. Biochim Biophys Acta Mol Cell Res. 2021;1868:119104.
- [CrossRef] [PubMed] [Google Scholar]
- Action and therapeutic targets of myosin light chain kinase, an important cardiovascular signaling mechanism. Pharmacol Res. 2024;206:107276.
- [CrossRef] [PubMed] [Google Scholar]
- Prevotella melaninogenica disrupted oral epithelial barrier function via myosin light chain kinase. Oral Dis. 2024;30:5102-12.
- [CrossRef] [PubMed] [Google Scholar]
- Salmonella manipulates macrophage migration via SteC-mediated myosin light chain activation to penetrate the gut-vascular barrier. EMBO J. 2024;43:1499-518.
- [CrossRef] [PubMed] [Google Scholar]
- SIK2 promotes ovarian cancer cell motility and metastasis by phosphorylating MYLK. Mol Oncol. 2022;16:2558-74.
- [CrossRef] [PubMed] [Google Scholar]
- The SNHG12/microRNA-15b-5p/MYLK axis regulates vascular smooth muscle cell phenotype to affect intracranial aneurysm formation. Microvasc Res. 2024;152:104643.
- [CrossRef] [PubMed] [Google Scholar]
- MYLK and CALD1 as molecular targets in bladder cancer. Medicine (Baltimore). 2023;102:e36302.
- [CrossRef] [PubMed] [Google Scholar]
- Myosin light-chain kinase inhibition potentiates the antitumor effects of avapritinib in PDGFRA D842V-mutant gastrointestinal stromal tumor. Clin Cancer Res. 2023;29:2144-57.
- [CrossRef] [PubMed] [Google Scholar]
- Molecular classification and emerging targeted therapy in endometrial cancer. Int J Gynecol Pathol. 2020;39:26-35.
- [CrossRef] [PubMed] [Google Scholar]
- Adjuvant therapy for endometrial cancer in the era of molecular classification. J Midlife Health. 2024;15:142-52.
- [CrossRef] [PubMed] [Google Scholar]
- Single-cell transcriptomic analysis highlights origin and pathological process of human endometrioid endometrial carcinoma. Nat Commun. 2022;13:6300.
- [CrossRef] [PubMed] [Google Scholar]
- Endometrial cancer: An overview of pathophysiology, management, and care. Semin Oncol Nurs. 2019;35:157-65.
- [CrossRef] [PubMed] [Google Scholar]
- FOXP2 regulates thyroid cancer cell proliferation and apoptosis via transcriptional activation of RPS6KA6. Exp Ther Med. 2022;23:434.
- [CrossRef] [PubMed] [Google Scholar]
- Low expression of FOXP2 predicts poor survival and targets caspase-1 to inhibit cell pyroptosis in colorectal cancer. J Cancer. 2022;13:1181-92.
- [CrossRef] [PubMed] [Google Scholar]
- si-SNHG5-FOXF2 inhibits TGF-β1-induced fibrosis in human primary endometrial stromal cells by the Wnt/β-catenin signalling pathway. Stem Cell Res Ther. 2020;11:479.
- [CrossRef] [PubMed] [Google Scholar]
- Wogonoside alleviates colitis by improving intestinal epithelial barrier function via the MLCK/pMLC2 pathway. Phytomedicine. 2020;68:153179.
- [CrossRef] [PubMed] [Google Scholar]
- Recent advances in the development of Rho kinase inhibitors (2015-2021) Med Res Rev. 2024;44:406-21.
- [CrossRef] [PubMed] [Google Scholar]
- Excitable Rho dynamics control cell shape and motility by sequentially activating ERM proteins and actomyosin contractility. Sci Adv. 2024;10:eadn6858.
- [CrossRef] [PubMed] [Google Scholar]
- High extracellular glucose promotes cell motility by modulating cell deformability and contractility via the cAMP-RhoA-ROCK axis in human breast cancer cells. Mol Biol Cell. 2023;34:ar79.
- [CrossRef] [PubMed] [Google Scholar]
- Melatonin potentiates sensitivity to 5-fluorouracil in gastric cancer cells by upregulating autophagy and downregulating myosin light-chain kinase. J Cancer. 2023;14:2608-18.
- [CrossRef] [PubMed] [Google Scholar]
- Myosin light chain kinase is a potential target for hypopharyngeal cancer treatment. Biomed Pharmacother. 2020;131:110665.
- [CrossRef] [PubMed] [Google Scholar]