Translate this page into:
Newcastle disease virus promotes pyroptosis in medulloblastoma cells by regulating interferon-gamma-mediated guanylate-binding protein 1 expression and activating caspase-4
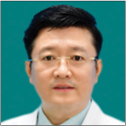
*Corresponding author: Kongbin Yang, Department of Neurosurgery, Fifth Affiliated Hospital, Guangzhou Medical University, Guangzhou, Guangdong, China. gzyangkongbin@163.com
-
Received: ,
Accepted: ,
How to cite this article: Ren P, Yu J, Wang D, Zeng L, Zhang X, Liu X, et al. Newcastle disease virus promotes pyroptosis in medulloblastoma cells by regulating interferon-gamma-mediated guanylate-binding protein 1 expression and activating caspase-4. CytoJournal. 2024;21:39. doi: 10.25259/Cytojournal_39_2024
Abstract
Objective:
The literature has reported that Newcastle disease virus (NDV) can have inhibitory effects on various tumors. This study aims to investigate the mechanism by which NDV induces pyroptosis in medulloblastoma (MB) cells.
Material and Methods:
We treated MB cell lines Daoy and D283 with NDV or recombinant interferon-gamma (IFN-g) proteins. Guanylate-binding proteins (GBPs) were measured using real-time quantitative polymerase chain reaction. Small interfering RNA-specific targeting GBP1 was transfected into MB cells. Apoptosis was assessed using Terminal deoxynucleotidyl transferase-mediated deoxyuridine triphosphate nucleoside nick end labeling and flow cytometry assays. Pyroptosis-related proteins, including caspase-4, caspase-1, and gasdermin D (GSDMD), were detected using Western blotting.
Results:
Bioinformatics analysis revealed that GBP family genes and interferon-related genes might be responsive to NDV stimulation in MB cells. Treatment with NDV resulted in increased IFN-g levels and upregulated GBP expression, particularly GBP1. In addition, IFN-g treatment induced GBP1 expression and enhanced cell apoptosis. GBP1 knockdown attenuated the decreased cell proliferation and increased cell apoptosis induced by NDV in MB cells. GBP1 overexpression upregulated the expression of pyroptosis-related proteins, including caspase-4, caspase-1, and GSDMD, subsequently leading to inhibition of cell proliferation and an increase in cell apoptosis levels. The silencing of caspase-4 confirmed the regulatory role of GBP1 in MB cell pyroptosis.
Conclusion:
Our findings suggest that NDV elevates IFN-g and GBP1 expression in MB cells, potentially contributing to caspase-4-mediated pyroptosis activation.
Keywords
Newcastle disease virus (NDV)
Pyroptosis
Interferon-gamma
Caspase-4
INTRODUCTION
Medulloblastoma (MB) is a highly malignant intracranial tumor, accounting for approximately 60% of all pediatric embryonal tumors. The complexity of its pathogenesis, the lack of effective treatment, and prognostic uncertainty make MB an urgent challenge to overcome.[1] As molecular biology, genetics, and immunology have advanced, our understanding of MB has deepened, and the treatment methods have expanded. However, the high recurrence rate and low survival rate of MB remain serious problems.[2] Therefore, this study aimed to explore a new treatment for MB, specifically the Newcastle disease virus (NDV).
As a highly infectious agent of avian disease, NDV has attracted widespread attention in cancer therapy. By utilizing its selective replication and lysis of tumor cells, NDV exhibits potential anti-tumor effects.[3] Furthermore, NDV is anticipated to become a new type of biotherapy in cancer treatment, offering safer and more effective treatment options to patients with cancer. In addition, with the continuous development of genetic engineering technology, NDV can be genetically modified to enhance its anti-tumor effect and reduce side effects.[4] Meng et al. modified NDV to express an angiogenic inhibitor, which exerts an enhanced therapeutic efficacy in the colon cancer model.[5] However, NDV has different effects on various tumors.[6] Herein, we attempted to examine the killing effect and molecular mechanism of NDV on MB cells.
The interaction between NDV and the host immune system, especially their ability to induce interferon (IFN) production, may be one of the mechanisms by which NDV kill tumor cells.[7] Production of IFN can be induced by NDV, thereby activating the host immune system to change the tumor microenvironment.[8] As an essential antiviral protein, IFN has broad antiviral, anti-tumor, and immune regulatory functions. Meister et al. reported that IFN-gamma (IFN-g)-mediated restoration of caspase-8 in MB cells might enhance apoptotic pathways relevant to chemotherapy and radiotherapy response.[9] Weng et al. reported that IFN-b induced MB cell apoptosis by activating extracellular signal-regulated kinase 2 and p38 mitogen-activated protein kinase signaling pathway.[10] Investigating whether NDV mediates MB cell apoptosis through IFN is worthwhile.
Guanylate-binding proteins (GBPs) are characterized by their ability to specifically bind guanine nucleotides, which regulate membrane, cytoskeleton, and cell cycle progression dynamics.[11] Abnormal expression of GBPs is closely correlated with cancer. Zhao et al. reported that GBP1 expression can be a predictive biomarker for immunotherapy.[12] Moreover, GBP1 expression is strongly provoked by IFN-g and restrains cellular proliferation during inflammation.[13] Besides, GBP2 is associated with poor survival and malignancy in clear-cell renal cell carcinoma.[14] Furthermore, there is a close relationship between GBP expression and viral infection.[15,16] Hence, we hypothesized that NDV may inhibit MB cells through IFN-activated GBPs. In this study, we are the first to use NDV to stimulate MB cells and explore their mechanisms.
MATERIAL AND METHODS
Database analysis
To investigate the effect of NDV on gene expression in MB cells, a sequencing dataset (GSE227791, https://www.ncbi.nlm.nih.gov/geo/query/acc.cgi?acc=GSE227791) for the NDV-infected glioma cell line LN229 was reanalyzed with GEO2R method. The differentially expressed genes were visualized as volcano plot.
Cell culture
Two MB cell lines – Daoy (Cat: HTB-186) and D283 (Cat: HTB-185) cells – were obtained from the American Type Culture Collection. Daoy cells were cultured in Dulbecco’s modified Eagle medium (DMEM) (Cat: E600003, Sangon Biotech, Shanghai, China), whereas D283 cells were cultured in the DMEM/F12 medium (Cat: PM150312B, Pricella, Wuhan, China). Cell mycoplasma and STR testing was performed to ensure the availability of cell lines. Both media were supplemented with 10% fetal bovine serum (Cat: A5256701, GIBCO BRL, Grand Island, NY, USA) and 1% penicillin/streptomycin (Cat: B540734, Sangon Biotech). Cells were cultured at 37°C and 5% carbon dioxide (CO2) in a Thermo Scientific™ BB 150 CO2 incubator (Thermo Fisher Scientific, Waltham, MA, USA).
Cell transfection
To intervene in the expression of GBP1 in cancer cells, the coding sequence of GBP1 was cloned and recombined into the pcDNA3.1 plasmid (Cat: V79520, Invitrogen, Carlsbad, CA, USA). The small interfering RNA specifically targeting GBP1 (siRNA: 5’- UUUUUGACUGGUACCUUUCUU -3’) and caspase-4 (siRNA: 5’- UGUUAAAAAAGGUUUGAAGAA -3’) was synthesized using (Genechem Co., Ltd., Shanghai, China). The siRNA and the pcDNA3.1 plasmid were transfected into Daoy or D283 cells according to the instructions on the Lipofectamine 2000 kit (Cat: 11668500, Invitrogen).
Cell treatment
The NDV (D90 strain) was provided by the Harbin Veterinary Research Institute, Chinese Academy of Agricultural Sciences (Harbin, China). To explore the inhibitory effect of NDVs, the MB cells grown to 80% density were inoculated with NDVs (1 MOI) at 37°C. After 1 h of incubation, the supernatant was removed to clean unattached viruses. Subsequently, the cells were washed thrice and cultured in a maintenance medium. As the NDV may inhibit MB cells by promoting IFN secretion, we stimulated MB cells with IFN-g recombinant protein (100 ng/L, for 48 h, Cat: IF002, Merck Millipore, Billerica, MA, USA).
Cell activity detection
We seeded MB cells (1 × 104) in a 96-well plate containing 100 μL of maintenance medium in each hole to measure cell activity. After grouping processing, we removed the culture medium from each well and added 100 μL of pre-configured cell counting kit-8 solution (Cat: C0038, Beyotime Biotechnology, Shanghai, China). After incubation in the cultivation chamber for 1 h, the plate was transfected to a microplate reader (Thermo Fisher Scientific) to detect the optical density at 450 nm wavelength.
Apoptosis detection
Apoptosis was measured using Terminal deoxynucleotidyl transferase -mediated deoxyuridine triphosphate nucleoside nick end labeling (TUNEL, Cat: C1090, Beyotime Biotechnology). The fixed cells (1 × 106) in a 6-well plate were rapidly treated with TritonX-100 for 8 min. After washing, we added 500 μL of the pre-prepared TUNEL reaction mixture to each well and incubated them for 1 h in a dark and humid box at 37°C. The cell nucleus was stained with 3, 3’-diaminobenzidine, and the stained cells (red) were observed under a fluorescence microscope (Olympus, Tokyo, Japan).
Furthermore, apoptosis was detected using a flow cytometry assay with an Annexin V-FITC/PI detection kit (Cat: C1065M, Beyotime Biotechnology). After the cells were digested with trypsin, we added 195 μL of Annexin V-FITC binding buffer and gently resuspended the cells (2.5 × 105). We added 5 μL Annexin V- Fluorescein Isothiocyanate solution and 10 μL propidium iodide staining solution and incubated at 20 ~ 25°C for 15 min in the dark. The fluorescence signals were quantified using a BD FACSCantoII flow cytometer (BD Biosciences, San Jose, CA, USA).
Reverse transcription polymerase chain reaction (PCR)
Total RNA was extracted using TRIzol (Cat: 15596018CN, Invitrogen), and its concentration was measured using a NanoDrop microspectrophotometer (Thermo Fisher Scientific). A total of 1 μg of RNA was used as a template for reverse transcription by using the BeyRT II cDNA First Chain Synthesis Kit (Cat: D7168M, Beyotime Biotechnology). Real-time quantitative PCR (qPCR) was performed using SYBRGreen qPCR Mix (Cat: D7510, Beyotime Biotechnology) in an ABI 7500 system (Applied Biosystems, Carlsbad, CA, USA). Primers targeting GBP1, GBP2, GBP3, GBP4, and GBP5 were synthesized using Sangon Biotech (Shanghai, China). Table S1 shows the sequence. The gene 3-phosphate dehydrogenase (GAPDH) was utilized as an internal reference. The reaction process includes 94°C for 2 min, 94°C for 20 s, 58°C for 20 s, 72°C for 20 s, and 40 cycles. The 2–ΔΔCt method was used to quantify gene expression levels.
Western blotting method
Total protein was collected using radioimmunoprecipitation assay lysis buffer (Cat: R0010, Solarbio, Beijing, China) and quantified by a bicinchoninic acid protein assay kit (Cat: P0011, Beyotime Biotechnology). Protein samples were separated on an 8% or 12% sodium dodecyl sulfatepolyacrylamide gel electrophoresis gel using electrophoresis and transferred to a polyvinylidene fluoride membrane. After incubation with a 5% skim milk powder solution for 1 h, the membrane was incubated with primary antibodies, anti-GBP1 (ab252832, 1:1000 diluted, Abcam, Cambridge, MA, USA), caspase-4 (ab238124, 1:1000 diluted), caspase-1 (ab179515, 1:1000 diluted), GSDMD-N (ab215203, 1:1000 diluted), and anti-GAPDH (ab8245, 1:5000 diluted), followed by horseradish peroxidase-labeled immunoglobin G secondary antibody (ab6721, 1:10000 diluted). After washing and color reaction with enhanced chemiluminescence solution (Cat: PE0010, Solarbio), the target protein appeared as visible bands on the membrane and transfer it to x-ray film (Cat: FF082, Beyotime Biotechnology).
Immunofluorescence
GBP1 expression in MB cells after stimulation with NDV was measured using an immunofluorescence assay. The cells were collected and fixed with 4% paraformaldehyde in phosphate-buffered saline for 15 min and washed thrice with phosphate-buffered saline with Tween 20. After permeabilization with triton X-100 and blocking with 5% bovine serum albumin, cells were incubated with anti-GBP1 antibody (ab252832, 1:500 diluted, and Abcam), followed by washing and incubation with secondary antibodies (ab130798, Abcam). To stain the cell nucleus, the 4’,6-diamidino-2-phenylindole (DAPI, Cat: C1002, Beyotime Biotechnology) was added and incubated in the dark for 15 minutes. The fluorescence signal was observed under a fluorescence microscope (Olympus). Six images with a random field of view were obtained and used for quantitative analysis of GBP1 expression. The images were treated with Image-Pro Plus 6.0 software (Media Cybernetics, MD, USA), and the optical density value of the positive signal was determined and considered as the relative expression level of GBP1.
Enzyme-linked immunosorbent assay (ELISA)
The release of lactate dehydrogenase (LDH) can reflect cellular damage, while interleukin (IL)-18 and IL-1b are inflammatory factors associated with caspase-1 protein during cell pyroptosis. Hence, the LDH, IFN-a, IFN-g, IL-18, and IL-1b concentrations in MB cell culture supernatant were detected using ELISA. The process was performed according to the instructions on the LDH, IFN-a, IFN-g, IL-18, or IL-1b ELISA detection kit (Cat: BC0685, SEKH-0045, SEKH-0046, SEKH-0028, SEKH-0002, Solarbio).
Statistic analysis
All values are expressed as the mean ± standard error of the mean. Statistical analysis was performed with the unpaired t-test for two groups or one-way analysis of variance (GraphPad Prism software, Version 8.0.1.244; La Jolla, CA, USA) followed by a post-Tukey test for multiple groups with all data points showing a normal distribution. All experiments were independently repeated 3 times. P < 0.05 was considered statistically significant.
RESULTS
Expression of GBP family in NDV-infected MB
A sequencing dataset (GSE227791) for the NDV-infected glioma cell line LN229 discovered many differentially expressed genes. Figure 1a displays that many GBP family genes (GBP1, GBP3, GBP4, and GBP5) and IFN-related genes (IRF1, IFIT3, IFIT5, ISG20, IFNB1, IFNL1, IFNL2, IFNL3, and IFNA7) were significantly upregulated after NDV infection (log2 [fold change] >2). Considering the role of IFN in viral infections and the relationship between GBP family genes and IFN activation pathways, we detected the expression of GBP family genes and two IFN-related genes (IFNB1 and IFNA7) in NDV-infected MB cells. After infection, the cell activity was significantly reduced at 48 h [Figure 1b]. The level of IFN-a and IFN-g in the supernatant was significantly increased in the NDV group [Figure 1c]. The mRNA expressions of IFNB1, IFNA7, GBP1, GBP4, and GBP5 were significantly increased in Daoy and D283 cells after NDV infection, while GBP2 and GBP3 did not differ significantly in D283 cells [Figure 1d and e]. The increased expression level of GBP1 protein was further expressed using Western blotting and immunofluorescence assay [Figure 1f and g]. Thus, NDV-induced decreases in cell activity may be associated with IFN-mediated pathways and abnormal expression of GBP family genes.
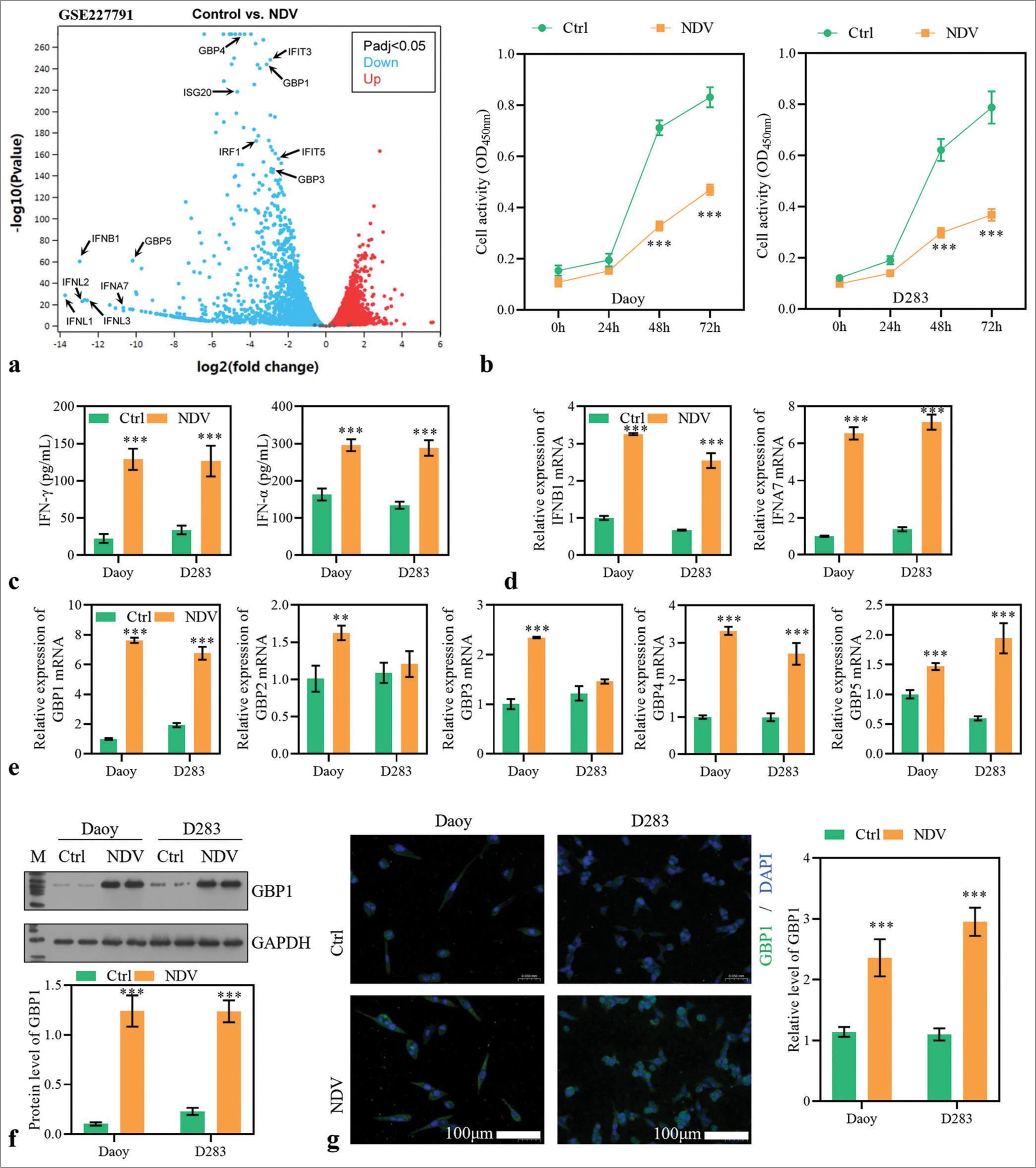
- Newcastle disease virus (NDV) infection changed the expression of guanylate-binding protein (GBPs) in medullobl astoma (MB) cells. (a) A sequencing dataset (GSE227791) for NDV rLa Sota-GFP strain infected glioma cell line LN229 discovered many differentially expressed genes. (b) Daoy and D283 cells were treated with NDV. Cell activity was measured with cell counting kit-8. (c) Interferon (IFN)-gamma and IFN-a levels in the supernatant. (d) The expressions of IFNB1 and IFNA7 were detected using reverse transcription polymerase chain reaction (RT-PCR) in MB cells 48 h after virus infection. (e) The expressions of GBP1, GBP2, GBP3, GBP4, and GBP5 were detected using RT-PCR. (f and g) The protein level of GBP1 was assessed using Western blotting method and immunofluorescence assay. (IFNL1~3: interferon lambda 1~3, IFNB1: interferon beta 1, IFNA7: interferon alpha 7, GBP1~5: guanylate binding protein 1~5, IFIT3, 5: interferon induced protein with tetratricopeptide repeats 3, 5, IRF1: interferon regulatory factor 1, ISG20: interferon stimulated exonuclease gene 20, Ctrl: Control, NDV: Newcastle disease virus. **P < 0.01, ***P < 0.001).
IFN-g stimulation activated GBP1 expression
To investigate the effect of IFN-mediated pathways on GBP1 expression, Daoy and D283 cells were stimulated with recombinant IFN-g proteins. Results found that the cell activity was significantly inhibited by IFN-g stimulation at 48 h [Figure 2a]. Moreover, TUNEL staining and flow cytometry assay revealed that IFN-g stimulation significantly promoted the MB cells’ apoptosis [Figure 2b and c]. Notably, mRNA and protein expression levels of GBP1 were increased in IFN-g-stimulated cells [Figure 2d-f]. Furthermore, pyroptosis-related proteins, including caspase-4, GSDMD-N, and cleaved caspase-1, were significantly upregulated by IFN-g stimulation [Figure 2e]. Therefore, it can be inferred that IFN-g stimulation activated GBP1 expression and the MB cell’s pyroptosis.
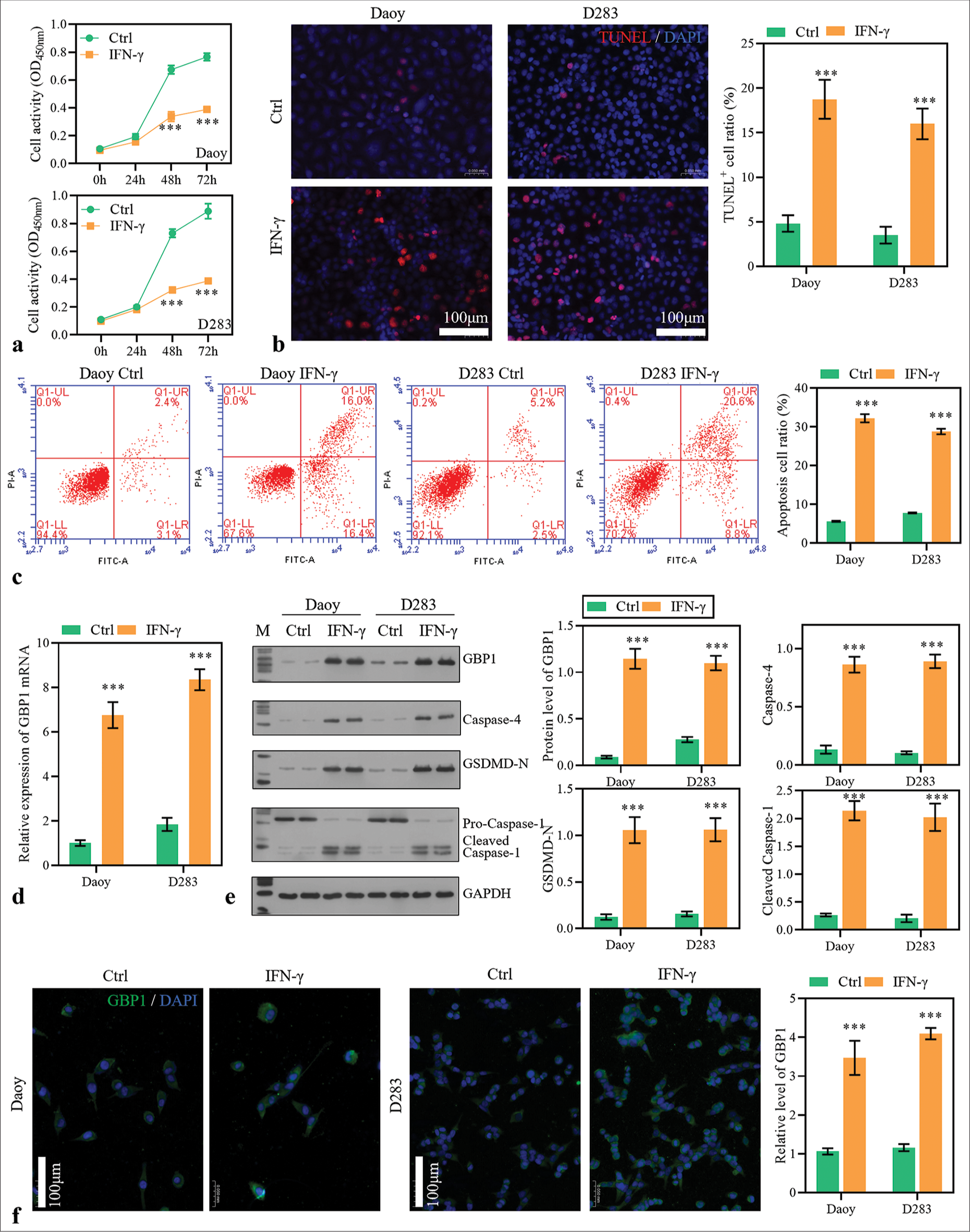
- Stimulation with interferon-gamma (IFN-g) activated guanylate-binding protein (GBP)1 expression and pyroptosis. (a) Medulloblastoma (MB) cells were stimulated with IFN-g recombinant protein (100 ng/L), and cell activity was measured with cell counting kit-8. (b and c) The apoptosis of MB cells 48 h after virus infection was measured by TdT-mediated dUTP nick end labeling and flow cytometry assay. (d) GBP1 expression was detected using reverse transcription polymerase chain reaction. (e) The protein levels of GBP1, caspase-4, gasdermin D (GSDMD)-N, and caspase-1 were detected using Western blotting method. (f) The protein level of GBP1 detected using immunofluorescence assay. ***P < 0.001.
GBP1 knockdown reduced cytotoxicity of NDV to MB cells
To verify whether GBP1 is involved in NDV inhibition of MB cells, GBP1 knockdown was performed in NDV-infected MB cells. After 24 h of transfection, the mRNA level of GBP1 was significantly downregulated [Figure 3a]. The decreased cell activity induced by NDV was partially reversed in silencing GBP1 group [Figure 3b]. The increased expression of caspase-4, GSDMD-N, and cleaved caspase-1 induced by NDV was partially reduced by GBP1 knockdown [Figure 3c], and the apoptosis rate [Figure 3d-3f]. In addition, LDH, IL-18, and IL-1b levels in the supernatant were significantly decreased by GBP1 knockdown [Figure 3g]. These results suggested that GBP1 was involved in the inhibitory action of NDV on MB cells.
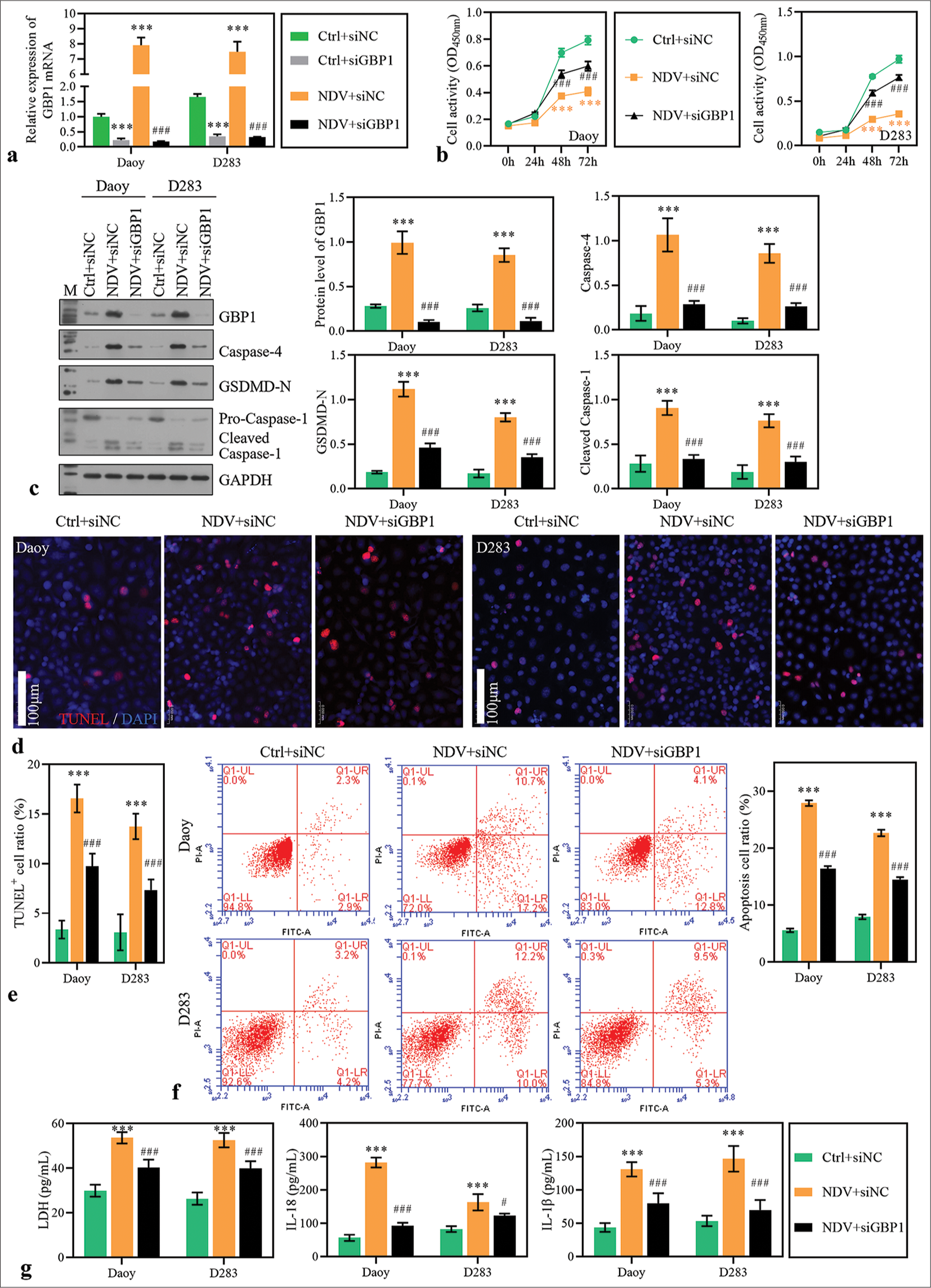
- Guanylate-binding protein (GBP)1 knockdown alleviated Newcastle disease virus (NDV)-induced pyroptosis. Daoy and D283 cells were transfected with small interfering RNA targeting GBP1 and treated with NDV. (a) GBP1 expression was detected using reverse transcription polymerase chain reaction in medulloblastoma (MB) cells 48 h after treatment. (b) Cell activity was measured with cell counting kit-8. (c) The protein levels of GBP1, caspase-4, gasdermin D (GSDMD)-N, and caspase-1 were detected using Western blotting method. (d-f) The apoptosis of MB cells 48 h after treatment was measured using TdT-mediated dUTP nick end labeling and flow cytometry assay. (g) Lactate dehydrogenase, interleukin (IL)-18, and IL-1b levels in the supernatant. ***P < 0.001, versus Ctrl + siNC group; #P < 0.05, ###P < 0.001, versus NDV + siNC group.
GBP1 overexpression promoted the pyroptosis of MB cells
To investigate the function of GBP1, its overexpression was performed in MB cells [Figure 4a]. GBP1 overexpression significantly decreased the cell activity of MB cells [Figure 4b] and significantly increased the protein levels of caspase-4, GSDMD-N, and cleaved caspase-1 [Figure 4c]. Moreover, it promoted MB cell apoptosis [Figure 4d and e]. The LDH, IL-18, and IL-1b levels in the supernatant significantly increased GBP1 overexpression [Figure 4f]. We hypothesized that GBP1 may function as a cancer suppressor gene in MB cells by inducing pyroptosis.
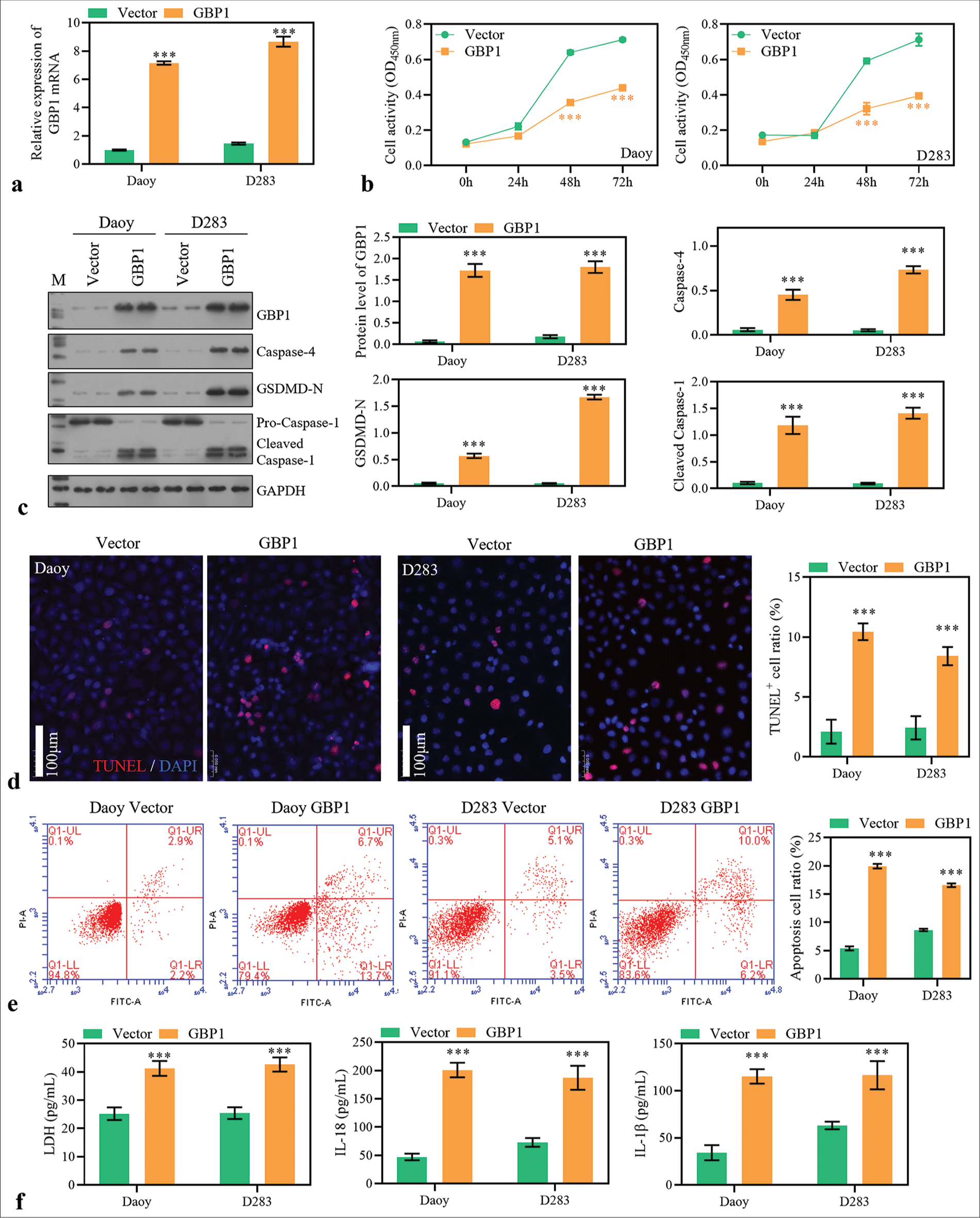
- Guanylate-binding protein (GBP)1 overexpression promotes medulloblastoma (MB) cell pyroptosis. (a) GBP1 expression was detected using reverse transcription polymerase chain reaction in MB cells 48 h after transfection. (b) Cell activity was measured with cell counting kit-8. (c) The protein level of GBP1, caspase-4, GSDMD-N, and caspase-1 was detected using Western blotting method. (d and e) The apoptosis of MB cells 48 h after transfection was measured using TdT-mediated dUTP nick end labeling and flow cytometry assay. (f) Lactate dehydrogenase, interleukin (IL)-18, and IL-1b levels in the supernatant. ***P < 0.001.
Silencing caspase-4 weakened the inhibitory effect of NDV on MB cells
To validate whether NDV activates the pyroptosis of MB cells, we silenced caspase-4 expression with siRNA in NDV-treated cells. Results of qPCR showed that the mRNA level of caspase-4 was significantly decreased after transfection [Figure 5a]. The deceased cell activity induced by NDV was partially reversed in the silencing caspase-4 group [Figure 5b]. Besides, the increased expression of GSDMD-N and cleaved caspase-1 induced by NDV was partially reduced by GBP1 knockdown [Figure 5c], and the apoptosis rate [Figure 5d-5f]. Furthermore, LDH, IL-18, and IL-1b levels in the supernatant were significantly decreased by caspase-4 knockdown [Figure 5g]. Results suggested that caspase-4-mediated pyroptosis was involved in the NDV-induced cancer cell-killing.
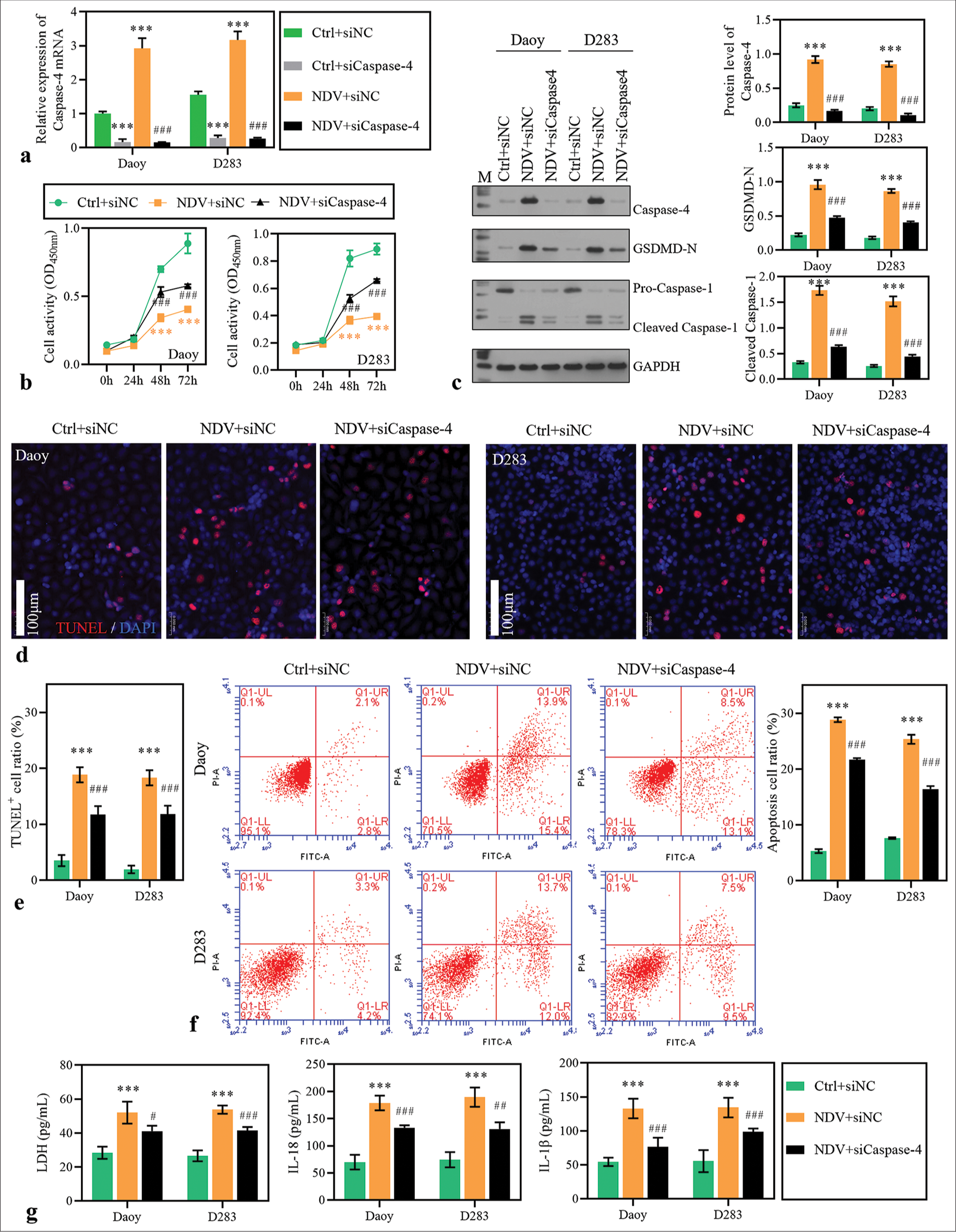
- Caspase-4 knockdown blocked Newcastle disease virus (NDV)-induced pyroptosis. Daoy and D283 cells were transfected with small interfering RNA targeting caspase-4 and treated with NDV. (a) Caspase-4 expression was detected using reverse transcription polymerase chain reaction in medulloblastoma (MB) cells 48 h after treatment. (b) Cell activity was measured with cell counting kit-8. (c) The protein levels of guanylate-binding protein 1, caspase-4, GSDMD-N, and caspase-1 were detected using Western blotting method. (d-f) The apoptosis of MB cells 48 h after treatment was measured using TdT-mediated dUTP nick end labeling and flow cytometry assay. (g) Lactate dehydrogenase, interleukin (IL)-18, and IL-1b levels in the supernatant. ***P < 0.001, versus Ctrl + siNC group; ##P < 0.01, ###P < 0.001, versus NDV + siNC group.
DISCUSSION
The application prospects of NDV in the treatment of tumors are extensive and full of challenges.[17] Yuan et al. reported that NDV activates methylation-related enzymes to reprogram m6A methylation in infected cells.[18] Therefore, the mechanism by which NDV kills tumor cells may involve multiple pathways. By reanalyzing sequencing data, we found that IFN-g-induced GBPs may be an important pathway in response to NDV infection. The increase in IFN-g level may be related to the immune response activated by NDV. Furthermore, NDV activates NK cells in pancreatic cancer and prevents cancer recurrence by promoting adaptive immunity.[19] However, our in vitro experiment revealed that the increase in IFN-g levels is independent of immune cells; after being stimulated by NDV, MB cells spontaneously upregulated IFNB1 and IFNA7 gene expression levels. We hypothesized that this may be because MB cell lines are very primitive and infinite cells, allowing them to retain some of the characteristics of stem cells. Zhu et al. also reported that herpes simplex virus-1 has the potential to stimulate IFN-g secretion in glioblastoma cells rather than T-cell secretion.[20] There are controversies on the role of IFN-g in MB cells. Lin et al. reported that IFN-g in the perinatal period can induce MB by the Sonic Hedgehog signaling pathway.[21] In addition, Zhou et al. reported that IFN-g promotes immune escape in gliomas by activating the epidermal growth factor receptor pathway.[22] However, Hotfilder et al. reported that IFN-g increased IL-6 production in human glioblastoma cell lines, which induces immune eradication of glioma cells.[23]
Herein, results showed that NDV induced the expression level of GBP1 in MB cells. Ma et al. reported that GBP1 is upregulated by treatment with IFN-g and infectious bronchitis virus (IBV) in chicken macrophage HD11 cells.[24] However, they found that GBP1 was pivotal in anti-IBV activity by degrading the IBV-N protein through the autophagy pathway. Our results suggested that high expression of GBP1 promotes caspase-4-mediated pyroptosis in MB cells. We recommend that the death effect induced by GBP1 targets the virus and the host. Some scholars believe that GBP1 may act as a double-edged sword in cancer. Furthermore, GBP1 can inhibit cancer cell proliferation and enhance their chemotherapy resistance. The previous studies reported that IFN-g-induced GBP1 inhibits mammary tumor growth in vivo.[25-27] Other previous studies reported that GBP1 plays a promoting role in glioblastoma,[28] lung adenocarcinoma,[29] and prostate cancer.[30] Tailor et al. reported that GBP1 overexpression enhanced the paclitaxel resistance of ovarian cancer through boosted cellular proteasomal activity.[31]
Unlike the controversy surrounding the role of GBP1 in tumors, GBP1 can activate cell pyroptosis, which regulates various disease progressions. Wandel et al. reported that GBP1 acts as a pattern recognition receptor that directly connects to lipopolysaccharide.[32] Johns and Galam reported that GBP1 induced inflammatory caspase-4 and triggered the proinflammatory cytokine release.[33] Our results demonstrated that the non-classical pathway, caspase-4 mediated pyroptosis, can be activated by GBP1. Dickinson et al. reported that supplementation with recombinant polymerized GBP1 or GBP2 can enhance lipopolysaccharides-induced caspase-4 activation.[34] Fisch et al. previously reported that GBP1 plays a crucial role in cell death by regulating pyroptosis and apoptosis.[35] Recently, they reported that the viral protein TgIST can increase GBP1 activity by depleting PIM1.[36] Furthermore, PIM1 can modify GBP1 and cause its inactivation. In MB cells, we found that GBP1 transcription was activated by NDV-induced IFN-g stimulation. Notably, our results showed that the classical pathway of pyroptosis, caspase-1, was induced in the NDV infection group.[37] Because caspase-1 and caspase-4 protein levels changed after NDV stimulation, we chose to detect the Annexin V protein signal. In addition, our results displayed that the concentrations of LDH, IL-18, and IL-1b in MB cell culture supernatant were increased by NDV treatment, which proved that NDV induced cell damage and pyroptosis in MB cells. However, there is no evidence to suggest a correlation between NDV and caspase-1 activation. The accumulation of reactive oxygen species can activate caspase-1,[38] which may be a follow-up reaction to the caspase-4 pathway.
Although clinical trials utilizing NDV are limited, the NDV-induced activation of the immune system plays a significant role in oncolysis and the maintenance of long-lasting anti-tumor effects.[39] There are limitations to our study. First, cell apoptosis, rather than cell pyroptosis, was detected. Cell apoptosis primarily relies on exogenous cytokine stimulation and endogenous mitochondrial pathways. Cell apoptosis and pyroptosis are similar in their reliance on activating caspase family proteins.[40] Second, there is a lack of in vivo animal experiments to confirm the relevant results. Modifying NDV and conducting in vivo animal experiments may promote the clinical application of NDV in MB. In addition,with the promotion and application of multi-omics technology and analysis,[41] the mechanism of NDV inhibiting tumor will be clearer.
SUMMARY
We propose that NDV promotes IFN-g and GBP1 expression and induces cell apoptosis in MB cells. With the continuous innovation of genetic modification technology, NDV treatment for tumors will become increasingly precise. In addition, IFN-g might activate the caspase-4-mediated pyroptosis in MB cells by regulating GBP1 expression. Furthermore, GBP family proteins may be essential targets in future cancer treatment.
AVAILABILITY OF DATA AND MATERIALS
The datasets used in this study are available from the corresponding author on reasonable request.
ABBREVIATIONS
NDV - Newcastle disease virus
MB – medulloblastoma
GBPs - Guanylate-binding proteins
IFN-g - interferon-gamma
IFN-a - interferon- alpha
IL-18 - interleukin-18
IL-1b - interleukin-1beita
GSDMD - gasdermin D
DMEM - Dulbecco’s modified Eagle medium
LDH - lactate dehydrogenase
AUTHOR CONTRIBUTIONS
KBY and PWR: Concepted and designed the research; JYY, XHL and YFC: Acquired the data; LJZ, XYZ and JYY: Analyzed and interpreted data; PWR and XQZ: Drafted the manuscript; DXW and ZJH: Revised manuscript for important intellectual content. All authors contributed to this present work. All authors read and approved the manuscript.
ETHICS APPROVAL AND CONSENT TO PARTICIPATE
This study did not involve direct research with human or animal samples and therefore does not require ethics committee approval.
CONFLICT OF INTEREST
The authors declare no conflict of interest.
EDITORIAL/PEER REVIEW
To ensure the integrity and highest quality of CytoJournal publications, the review process of this manuscript was conducted under a double-blind model (authors are blinded for reviewers and vice versa) through an automatic online system.
Supplementary material associated with this article can be found:
https://dx.doi.org/10.25259/Cytojournal_39_2024
FUNDING
This project was supported by Guangzhou City Science and Technology Plan Project, Guangdong Province, China (No.2023A03J0822, Kongbin Yang), Guangdong Provincial Basic and Applied Basic Research Fund Project-Provincial Enterprise Joint Fund, Guangdong Province, China (No.2021A1515220097, Xiaoyong Zhao).
References
- Medulloblastoma: WHO 2021 and beyond. Pediatr Dev Pathol. 2022;25:23-33.
- [CrossRef] [Google Scholar]
- Clinical trials in high-risk medulloblastoma: Evolution of the SIOP-Europe HR-MB trial. Cancers (Basel). 2022;14:374.
- [CrossRef] [Google Scholar]
- Oncolytic Newcastle disease virus as a prospective anti-cancer therapy. A biologic agent with potential to break therapy resistance. Expert Opin Biol Ther. 2015;15:1757-71.
- [CrossRef] [Google Scholar]
- Oncolytic newcastle disease virus co-delivered with modified PLGA nanoparticles encapsulating temozolomide against glioblastoma cells: Developing an effective treatment strategy. Molecules. 2022;27:5757.
- [CrossRef] [Google Scholar]
- Newcastle disease virus expressing an angiogenic inhibitor exerts an enhanced therapeutic efficacy in colon cancer model. PLoS One. 2022;17:e0264896.
- [CrossRef] [Google Scholar]
- Newcastle disease virus: a promising agent for tumour immunotherapy. Clin Exp Pharmacol Physiol. 2012;39:725-730.
- [CrossRef] [Google Scholar]
- Oncolytic newcastle disease virus as cutting edge between tumor and host. Biology (Basel). 2013;2:936-75.
- [CrossRef] [Google Scholar]
- Counteracting immunosuppression in the tumor microenvironment by oncolytic newcastle disease virus and cellular immunotherapy. Int J Mol Sci. 2022;23:13050.
- [CrossRef] [Google Scholar]
- Interferon-gamma mediated up-regulation of caspase-8 sensitizes medulloblastoma cells to radio-and chemotherapy. Eur J Cancer. 2007;43:1833-41.
- [CrossRef] [Google Scholar]
- Anti-apoptotic activity of Japanese encephalitis virus NS5 protein in human medulloblastoma cells treated with interferon-β. J Microbiol Immunol Infect. 2018;51:456-64.
- [CrossRef] [Google Scholar]
- Human guanylate-binding proteins in intracellular pathogen detection, destruction, and host cell death induction. Curr Opin Immunol. 2023;84:102373.
- [CrossRef] [Google Scholar]
- Guanylate-binding protein 1 as a potential predictor of immunotherapy: A Pan-cancer analysis. Front Genet. 2022;13:820135.
- [CrossRef] [Google Scholar]
- Guanylate-binding protein 1: An emerging target in inflammation and cancer. Front Immunol. 2020;10:3139.
- [CrossRef] [Google Scholar]
- Guanylate-binding protein 2 expression is associated with poor survival and malignancy in clear-cell renal cell carcinoma. Anticancer Res. 2022;42:2341-54.
- [CrossRef] [Google Scholar]
- Guanylate-binding protein 2 exerts GTPase-dependent anti-ectromelia virus effect. Microorganisms. 2023;11:2258.
- [CrossRef] [Google Scholar]
- Guanylate-binding protein 1 inhibits nuclear delivery of pseudorabies virus by disrupting structure of actin filaments. Vet Res. 2023;54:21.
- [CrossRef] [Google Scholar]
- Oncolytic Newcastle disease virus for cancer therapy: Old challenges and new directions. Future Microbiol. 2012;7:347-67.
- [CrossRef] [Google Scholar]
- Newcastle disease virus activates methylation-related enzymes to reprogram m(6)A methylation in infected cells. Vet Microbiol. 2023;281:109747.
- [CrossRef] [Google Scholar]
- Newcastle disease virus mediates pancreatic tumor rejection via NK cell activation and prevents cancer relapse by prompting adaptive immunity. Int J Cancer. 2017;141:2505-16.
- [CrossRef] [Google Scholar]
- Enhancement of CD70-specific CAR T treatment by IFN-γ released from oHSV-1-infected glioblastoma. Cancer Immunol Immunother. 2022;71:2433-48.
- [CrossRef] [Google Scholar]
- Interferon-gamma induced medulloblastoma in the developing cerebellum. J Neurosci. 2004;24:10074-83.
- [CrossRef] [Google Scholar]
- The crosstalk between the EGFR and IFN-γ pathways and synergistic roles in survival prediction and immune escape in gliomas. Brain Sci. 2023;13:1349.
- [CrossRef] [Google Scholar]
- Interferon-gamma increases IL-6 production in human glioblastoma cell lines. Anticancer Res. 2000;20:4445-50.
- [Google Scholar]
- Guanylate-binding protein 1 restricts avian coronavirus infectious bronchitis virus-infected HD11 cells. Poult Sci. 2023;102:102398.
- [CrossRef] [Google Scholar]
- Interferon gamma-induced human guanylate binding protein 1 inhibits mammary tumor growth in mice. Mol Med. 2010;21:880-91.
- [CrossRef] [Google Scholar]
- GBP-1 acts as a tumor suppressor in colorectal cancer cells. Carcinogenesis. 2013;34:153-62.
- [CrossRef] [Google Scholar]
- IFN-γ-response mediator GBP-1 represses human cell proliferation by inhibiting the Hippo signaling transcription factor TEAD. J. 2018;475:2955-67.
- [CrossRef] [Google Scholar]
- Guanylate binding protein-1 mediates EGFRvIII and promotes glioblastoma growth in vivo but not in vitro. Oncotarget. 2016;7:9680-91.
- [CrossRef] [Google Scholar]
- Guanylate binding protein 1 (GBP-1) promotes cell motility and invasiveness of lung adenocarcinoma. Biochem Biophys Res Commun. 2019;519:266-72.
- [CrossRef] [Google Scholar]
- Oncogenic role of guanylate binding protein 1 in human prostate cancer. Front Oncol. 2020;9:1494.
- [CrossRef] [Google Scholar]
- Guanylate-binding protein 1 modulates proteasomal machinery in ovarian cancer. iScience. 2023;26:108292.
- [CrossRef] [Google Scholar]
- Guanylate-binding proteins convert cytosolic bacteria into caspase-4 signaling platforms. Nat Immunol. 2020;21:880-91.
- [CrossRef] [Google Scholar]
- Guanylate binding protein 1 (GBP1): A key protein in inflammatory pyroptosis. Cell Biochem Biophys. 2022;80:295-9.
- [CrossRef] [Google Scholar]
- LPS-aggregating proteins GBP1 and GBP2 are each sufficient to enhance caspase-4 activation both in cellulo and in vitro. Proc Natl Acad Sci U S A. 2023;120:e2216028120.
- [CrossRef] [Google Scholar]
- Human GBP1 is a microbe-specific gatekeeper of macrophage apoptosis and pyroptosis. EMBO J. 2019;38:e100926.
- [CrossRef] [Google Scholar]
- PIM1 controls GBP1 activity to limit self-damage and to guard against pathogen infection. Science. 2023;382:eadg2253.
- [CrossRef] [Google Scholar]
- Caspases in cell death, inflammation, and pyroptosis. Annu Rev Immunol. 2020;38:567-95.
- [CrossRef] [Google Scholar]
- Simvastatin induces pyroptosis via ROS/caspase-1/GSDMD pathway in colon cancer. Cell Commun Signal. 2023;21:329.
- [CrossRef] [Google Scholar]
- Fifty years of clinical application of newcastle disease virus: Time to celebrate! Biomedicines. 2016;4:16.
- [CrossRef] [Google Scholar]
- The caspase-3/GSDME signal pathway as a switch between apoptosis and pyroptosis in cancer. Cell Death Discov. 2020;6:112.
- [CrossRef] [Google Scholar]
- Survey on multi-omics, and multi-omics data analysis, integration and application. Current Pharmaceutical Analysis. 2023;19:267-281.
- [CrossRef] [Google Scholar]