Translate this page into:
Promotion of inflammatory response in mice with diabetes periodontitis: regulation of forkhead box protein M1 silencing to mediate activator protein-1 via reactive oxygen species production
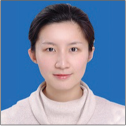
*Corresponding author: Yang Yang, State Key Laboratory of Oral Diseases and National Clinical Research Center for Oral Diseases, West China Hospital of Stomatology, Sichuan University, Chengdu, China. bzmwybzmwy@126.com
-
Received: ,
Accepted: ,
How to cite this article: Yang Y, Ren D, Peng B, Huang J, Yang B. Promotion of inflammatory response in mice with diabetes periodontitis: regulation of forkhead box protein M1 silencing to mediate activator protein-1 via reactive oxygen species production. CytoJournal. 2024;21:72. doi: 10.25259/Cytojournal_143_2024
Abstract
Objective:
Periodontitis, the sixth major complication of diabetes, has a mutually influential and mutually reinforcing relationship with diabetes. Forkhead box protein M1 (FOXM1) is essential for osteoclast differentiation, periodontitis, and diabetes. This study aims to investigate the potential action mechanisms and effects of FOXM1 on diabetes periodontitis.
Material and Methods:
A model of mice with diabetes periodontitis was created. The pathological damage of periodontal tissues in the mandibles of mice was assessed through hematoxylin–eosin staining. The expression of bone resorption-related, cell proliferation transcription, inflammatory, and oxidative stress-related factors in mice with diabetes periodontitis was analyzed through Western blot and quantitative reverse transcription polymerase chain reaction. Oxidative stress level was examined through enzyme-linked immunosorbent assay. Furthermore, plasmids with FOXM1 silencing were constructed using short hairpin RNA. The activity of activator protein-1 (AP-1) binding was measured via electrophoretic mobility shift assay. Reactive oxygen species (ROS) expression was detected using a dichlorodihydrofluorescein diacetate probe.
Results:
Treatment with the ROS inhibitor N-acetylcysteine ameliorated the degree of bone destruction, attenuated the inflammatory response, and mitigated oxidative stress in mice with diabetes periodontitis. Short hairpin-FOXM1 reduced the levels of FOXM1, increased the levels of C-Jun proto-oncogene and AP-1, enhanced AP-1 binding activity, and increased ROS production in the macrophage cells. sh-FOXM1 increased the mRNA and protein levels of tartrate-resistant acid phosphatase (TRAP), osteoclast-associated receptor (OSCAR), and nuclear factor-activated T-cell c1 (NFATc1). By contrast, JUN-small interfering RNA decreased the mRNA and protein expression of TRAP, OSCAR, and NFATc1. The silencing of FOXM1-regulated ROS production mediated AP-1 activation to promote macrophage osteoclast differentiation.
Conclusion:
FOXM1 plays a key role in diabetes periodontitis, and this study provides theoretical support for the clinical prevention and treatment of diabetes periodontitis in humans.
Keywords
Forkhead box protein M1
Reactive oxygen species
Diabetic
Periodontitis
Inflammatory response
INTRODUCTION
Diabetes is a systemic metabolic disease characterized by high blood sugar.[1] Long-term exposure to high blood sugar exerts various adverse effects on the patient’s body.[2] Periodontitis is a chronic inflammation of periodontal supporting tissues caused by dental plaque and host immune reactions, and it typically presents as alveolar bone resorption, loss of attachment, loose teeth, and even tooth loss.[3] The national oral health survey in our country shows that the incidence of periodontal disease is extremely high (over 90%) in adults and seriously affects oral health.[4] Diabetes and periodontitis can mutually influence and exacerbate each other’s progression. The incidence of periodontitis in diabetic patients considerably increases, and advanced-glycation end products formed during high-blood-sugar periods can activate the inflammatory pathways in periodontitis, leading to exacerbated inflammation and periodontal destruction in diabetic patients.[5] Moreover, the incidence of diabetes among patients with periodontitis is almost twice that among patients without periodontitis.[6] The progression of periodontitis is related to the increase in glycosylated hemoglobin, and periodontal treatment can help control blood sugar, thereby reducing the incidence of glycosylated hemoglobin and diabetes-related complications.[7] Therefore, diabetes periodontitis treatment is an urgent issue that needs to be addressed in clinical practice and research.
Derived from molecular oxygen, a number of chemically reactive molecules form the category known as reactive oxygen species (ROS). These molecules are crucial signaling agents that regulate many cellular processes.[8] When the level of ROS is too high, oxidative stress in the body is stimulated, exacerbating the destruction of periodontal tissues.[9] Evidence shows the involvement of ROS in multiple cancer-associated processes, such as apoptosis, angiogenesis, metastasis, and inflammation.[10] Furthermore, research has indicated that ROS can trigger inflammatory mediators, such as nuclear factor κB, activator protein-1 (AP-1), hypoxia inducible factor-1α, growth factors, inflammatory cytokines, and chemokines.[11-13] AP-1 encompasses dimeric transcription factors formed by Jun, Fos, or activating transcription factor subunits, which collectively bind to a shared DNA site known as the AP-1-binding site.[14] The previous studies have shown that the excessive release of ROS within periodontal tissues can indirectly activate redox-sensitive nuclear transcription factors, such as AP-1, thus contributing to the escalation of inflammatory and immune processes.[15] However, the mechanism by which ROS production mediates the activation of AP-1 to promote diabetes periodontitis remains unclear.
Forkhead box protein M1 (FOXM1), an essential component of the forkhead box family, plays a pivotal role in the proliferation and differentiation of normal cells.[16] Scientists recently discovered through bone metabolism research that enhanced osteogenic differentiation in human periodontal ligament cells (HPDLCs) results from the inhibition of FOXM1 through small interfering RNA transfection or a FOXM1 inhibitor.[17] Moreover, large quantities of multinucleated osteoclasts, along with an elevated receptor activator for nuclear factor κB ligand (RANKL) to osteoprotegerin (OPG) ratio, were observed in RAW264.7 cells co-cultured with HPDLCs that were either transfected with FOXM1 or treated with a specific FOXM1 inhibitor.[17] These results suggest that FOXM1 can impede osteoclast differentiation. Furthermore, FOXM1 in the respiratory mucosa of chronic respiratory diseases can accelerate the aggregation of eosinophils and macrophages by activating interleukin (IL)-5, thereby inducing goblet cell metaplasia and exacerbating lung inflammation.[18] In addition, the generation of ROS mediates the important role of AP-1 in periodontitis.[19] However, the specific mechanism by which FOXM1 regulates the generation of ROS to mediate AP-1 and promote diabetes periodontitis is unclear and has not been reported.
In this study, a mouse model with diabetes periodontitis was created to investigate the effect of silencing FOXM1 on the degree of bone destruction, inflammatory response, oxidative stress, and osteoclast differentiation in diabetes periodontitis mice. The aim is to provide references for targeted therapy of diabetes periodontitis in humans.
MATERIAL AND METHODS
Animal rearing
Male Balb/C mice (20–22 g, 6–8 weeks in age) were acquired from Beijing Baiaosike Biomedical Technology Co., Ltd. They were housed in individually ventilated cages, with 4–6 mice per cage, under pathogen-free conditions in an environment maintained at 23°C ± 2°C with a 12 h light/dark cycle. The mice had unrestricted access to standard rodent diet and water before the commencement of the study. The mice were randomly divided into groups (n = 10) for the experiment.
Diabetes periodontitis model and treatment
A total of 30 mice were randomly allocated into three distinct groups: The control group (n = 10), the diabetes-periodontitis model (DM) group (n = 10), and the DM + ROS inhibitor N-acetylcysteine (NAC; S0077, Beyotime, Shanghai, China) group (n = 10). Each group was adaptively fed for 1 week. The control group received normal feeding, and the DM and DM + NAC groups were fed a high-fat diet for 4 weeks, followed by intraperitoneal injection of streptozotocin (S817944, MACKLIN, Shanghai, China) at a dose of 30 mL/kg. Measurements taken 1 week after injection showed blood glucose levels exceeding 16.7 mmol/L and 24 h urinary microalbumin levels exceeding 30 mg/L. Subsequently, the rats were anesthetized by inhalation of 3% isoflurane (132220, Karamay, Shanghai, China), and a 5–0 silk suture was ligated around the maxillary second molar for 2 weeks.
At this point, the experimental mice were successfully modeled for DM. The experimental mice in the DM + NAC group were administered intraperitoneal NAC injection (4 mL•kg−1•times−1) 3 times/day for 14 days. Then, anesthesia was provided by intraperitoneal injection of sodium pentobarbital (45 mg/kg; 20090512, SINOPHARM, Beijing, China). Cervical dislocation was performed manually, and euthanasia was achieved in approximately 8–15 s.
The animal experiments complied with ethical standards and principles of animal protection.
Hematoxylin–eosin (HE) staining
Mandible tissues from each mouse were extracted and weighed. Afterward, the mandible tissues were preserved in 4% paraformaldehyde (BL539A, Biosharp Shanghai, China) for 24 h, followed by paraffin embedding. Subsequently, the tissues were cut into 3 μm thick sections and subjected to H&E staining (G1120, Solarbio, Beijing, China) to assess periodontal tissues. The extent of tissue damage was observed under a light microscope (CX31, OPLYMPUS, Tokyo, Japan) at ×100 magnification and photographed. The extent of tissue damage was evaluated with Image-Pro® Plus 6.0 software (Media Cybernetics).
Enzyme-linked immunosorbent assay (ELISA)
After euthanasia, mice mandibles were obtained and centrifuged at 2,700 g for 15 min at 4°C to analyze periodontal tissue oxidative stress factors. The malondialdehyde (MDA), superoxide dismutase (SOD), catalase (CAT), and glutathione (GSH) levels in the periodontal tissue homogenates were assessed using commercially provided ELISA kits (YJ, YOYOBIO, Shanghai, China). ELISA samples were labeled using a multifunctional enzyme labeler (Varioskan LUX, Thermo Fisher, Waltham, MA, the USA). ELISA results were normalized.
Electrophoretic mobility shift assay (EMSA)
EMSA was used to detect the binding activity of AP-1. The process began with 30 min of incubation at room temperature using a biotin-labeled probe that included the AP-1 binding site and 10 mg of nuclear extracts. Then, the resulting protein–DNA complexes were separated by running them on 5% polyacrylamide gel in a × 0.5 Tris buffer ethylene diamine tetra-acetic acid solution (798681, Sigma-Aldrich, St. Louis, MO, the USA). The EMSA gel containing the protein–DNA complex was transferred to a positively charged nylon membrane. The membrane was irradiated for 5 min using a ultraviolet cross-linking detector (EUV002, Beyotime, Shanghai, China) at a distance of 10 cm from the membrane. Afterward, the biotin-labeled DNA was analyzed using a chemiluminescent detection kit, which was obtained from Pierce (20148, Rockford, IL, the USA) and branded as the Light Shift Chemiluminescent EMSA kit.
Cell culture transfections
RAW264.7 cells derived from mouse mononuclear macrophages were obtained from Wuhan Procell Life Technology Co., Ltd. (CL-0190, Wuhan, China) and grown in Dulbecco’s modified Eagle medium (Sigma-Aldrich, St. Louis, MO, the USA) enriched with 10% (v/v) fetal bovine serum (Gibco, Carlsbad, CA, the USA), 100 U/mL penicillin, and 100 ug/mL streptomycin (Gibco, Carlsbad, CA, the USA). The mouse mononuclear macrophage RAW264.7 cells were incubated under 37°C, 5% Carbon dioxide, and saturated humidity conditions and subjected to short tandem repeat identification and mycoplasma testing. The target sequences of FOXM1 shRNA were sh-FOXM1 and 5'-CTCTTCTCCCTCAGATATA-3'. After the cells were cultured to the logarithmic phase, they were divided into RANKL-lipopolysaccharide (LPS), sh-FOXM1-RANKLLPS, sh-FOXM1-RANKL-LPS-NAC, sh-FOXM1-RANKLLPS-NAC + nonsense-siRNA, and sh-FOXM1-RANKLLPS-NAC + JUN-siRNA groups. Macrophage activation in the RANKL-LPS group was transfected by LPS (1 μg/mL; LPS25, Sigma-Aldrich, St. Louis, MO, the USA) combined with RANKL-LPS, which was adopted for control. The cells in the sh-FOXM1-RANKL-LPS group were transfected with the adenovirus-packaged sh-FOXM1 plasmid (Shanghai HANBIO Biotechnology Co., Ltd.) and RANKL-LPS to construct the sh-FOXM1-RANKL-LPS macrophage. The cells in the sh-FOXM1-RANKL-LPS-NAC group were induced by NAC, sh-FOXM1, and RANKL-LPS for cell activation. The cells in the sh-FOXM1-RANKL-LPS-NAC + nonsense-siRNA group were transfected with nonsense-siRNA, sh-FOXM1, RANKL-LPS, and NAC. The cells in the sh-FOXM1-RANKL-LPS-NAC + JUN-siRNA group were transfected with JUN-si-RNA corresponding to the core subunit C-Jun in macrophage AP1 heterodimers, shFOXM1, RANKL-LPS, and NAC.
2’,7’-Dichlorodihydrofluorescein diacetate (DCFH-DA)
A DCFH-DA probe from Beyotime Biotechnology Co., Ltd. (Shanghai, China) was employed to detect ROS. Initially, macrophages were placed on 12-well plates, allowed to grow overnight, and activated using RANKL-LPS for 12 h. After this period, the DCFH-DA probe was diluted at a ratio of 1:1000 with a serum-free medium (N6040-1L, Solarbio, Beijing, China), and 1 mL of the diluted probe was added to each well for another 30 min of incubation. Afterward, the medium was discarded, and the cells were rinsed two times with the serum-free medium. The level of staining within the cells was examined using a fluorescence microscope (DM6B, Leica, Germany). In addition, ROS levels were determined using a fluorescence spectrophotometer (F-280, Gangdong, Tianjin, China).
Quantitative reverse transcription polymerase chain reaction
Mandibles tissues were fully ground with liquid nitrogen and sonicated to make a homogenate. At the same time, transfected cell lines (RAW264.7) were collected. The total RNA of the tissue specimens and cultured cells was isolated using the Trizol (15596026, Invitrogen, Mountain View, CA, the USA) method, and complementary DNA was synthesized by a reverse-transcription kit (11119ES60, Yeasen, Shanghai, China). Afterward, quantitative reverse transcription polymerase chain reaction (qRT-PCR) was performed using the SYBR Green Master Mix kit (A46110, ThermoFisher, Waltham, MA, the USA) and primers synthesized by Shanghai Sangon Bioengineering Co., Ltd. (Shanghai, China). The primers used for qRT-PCR are shown in Table 1. With β-actin expression as an internal reference, the relative expression levels of gene mRNA were analyzed by the 2−∆∆Ct method.
Primer name | Upstream (5’-3’) | Downstream (5’-3’) |
---|---|---|
β-Actin | GGGAAATCGTGCCGTGACAT | GCGGCAGTGGCCATCTC |
OPG | TGTGTCCCTTGCCCTGACTACTC | CTCGGTTGTGGGTGCGGTTG |
PANKL | GAGCGAAGACACAGAAGCACTACC | GAGCCACGAACCTTCCATCATAGC |
MCS-F | CCGCGGGACGTCCTTAAGCATA | GAATTCGGACACGGGCATCTCAC |
IL-1β | GAGAAGCTCTCCACCTCAATG | GCCGTCTTTCCATACACAGG |
IL-10 | ACCTGGTAGAAGTGATGCC | CAAGGAGTTGTTTCCGTTA |
iNOS | CTTCCGGGCAGCCTGTGAGACG | ATCCCCAGGTGTTCCCCAGGTAGG |
NFATc1 | CCCGTCCACATCTGTCCAT | CAAGTAACCGTGTAGCTGCACAA |
TRAP | TAGGTGCCAAGGTCAAAAGG | CATGTAACAGCCCCCTGTCT |
OSCAR | CGAAGGTTCTGGCTCCT | CCTGCTGTGCCAATCAC |
qRT-PCR: Quantitative reverse transcription polymerase chain reaction, OPG: Osteoprotegerin, PANKL: Receptor activator for nuclear factor κB ligand, MCS-F: Macrophage colony-stimulating factor, IL-1β: Interleukin-1β, IL-10: Interleukin-10, iNOS: Inducible nitric oxide synthetase, NFATc1: Nuclear factor-activated T-cells c1, TRAP: Tartrate-resistant acid phosphatase, OSCAR: Osteoclast-associated receptor, A: Adenosine, C: Cytosine, G: Guanine, T: Thymine
Western blot
Mandible tissues were fully ground with liquid nitrogen, and transfected cell lines (RAW264.7) were collected. The tissue specimens and cultured cells were separately added with radio-immunoprecipitation assay lysis buffer (P0013B, Beyotime, Beijing, China) and centrifuged to obtain the protein supernatant. After quantification using a bicinchoninic acid kit (P0009, Beyotime, Beijing, China), the isolated proteins were separated by sodium dodecyl– sulfate polyacrylamide gel electrophoresis and transferred to polyvinylidene difluoride (PVDF) membranes (IPVH00010, Millipore, Boston, MA, the USA). The PVDF membranes were blocked with skim milk for 1.5 h and incubated with primary antibodies (anti-RANKL [ab239607, 1:2,000], anti-Macrophage colony-stimulating factor (MCS-F) [ab99178, 1:5,000], anti-OPG [ab73400, 1:500], anti-FOXM1 [ab180710, 1:2,000], anti-C-Jun [ab31419, 1:5,000], anti-IL-1β [ab9722, 1:10,000], anti-IL-10 [ab34843, 1:5,000], anti-Inducible nitric oxide synthetase[1] [ab15323, 1:5,000], anti-nuclear factor-erythropoietin 2-related factor 2 [Nrf2; ab62352, 1:5,000], anti-hemeoxygenase-1 [HO-1; ab305290, 1:5,000], anti-LaminA [ab264322, 1:5,000], anti-β-actin [ab8227, 1:5,000], anti-nuclear factor-activated T-cell c1 [NFATc1] [ab25916, 1:5,000], and anti-tartrate-resistant acid phosphatase [TRAP] [ab65854, 1:5,000], all of which were obtained from Abcam [Cambridge, the UK], and anti-osteoclast-associated receptor [OSCAR] [HPA073996, 1:5000, Atlas Antibodies, Wuhan, China] and anti-AP1 [1:5,000, IPODIX, Wuhan, China]) on a shaker at room temperature for 3 h. Then, the membrane was incubated with a secondary antibody (ab7090, Abcam, Cambridge, the UK) for 1.5 h, developed with the enhanced chemiluminescence kit (32106, Pierce, Waltham, MA, the USA), and detected using a protein imaging system (CliNX, ChemiScope 6200, Shanghai Qinxiang Scientific Instrument Co., Ltd., Shanghai, China). The relative protein expression levels of Nrf2 were detected with LaminA as an internal reference. The internal reference for other proteins is β-actin. ImageJ software (https://imagej.nih.gov/ij/) was utilized to quantify protein levels in the Western blots obtained.
Statistical analysis
The results were expressed as mean ± standard deviation, and statistical analysis was performed with the Statistical Package for the Social Sciences version 22.0 software (IBM, Armonk, NY, the USA). The t-test was used to compare the two groups. In instances where the study compared more than two distinct groups, analysis of variance, followed by Tukey’s post hoc test, was utilized to assess the differences among groups. P < 0.05 denoted statistical significance. Each experiment was repeated biologically 3 times.
RESULTS
Inhibition of ROS-alleviated bone damage in mice with diabetes periodontitis
NAC plays an important role in reducing ROS levels and bone resorption in periodontitis.[20] To clarify the effects of ROS inhibition on diabetes periodontitis in mice, we used NAC in the subsequent experiments. The hematoxylineosin (HE) staining results showed that compared with the control group, the model group (DM) exhibited epithelial deficiency, rupture, disorder of periodontal ligament fibers, and inflammatory cell infiltration in periodontal tissue. ROS inhibitor NAC intervention alleviated the pathological damage to the periodontal tissue [Figure 1a]. Moreover, the protein expression of bone destruction factors RANKL and MCS-F was higher in the DM group than in the control group, but OPG in the DM group was lower than that in the control group. With NAC intervention, the control group had a lower protein expression of RANKL and MCS-F than the DM group, whereas OPG in the control group was higher than that in the DM group [Figure 1b-e]. The trend of mRNA expression of RANKL and MCS-F in the mouse jawbone was consistent with the trend of protein expression [Figure 1f-h]. The protein expression of FOXM1 in the DM group was markedly lower than that in the control group, and the expression of C-Jun and AP1 was considerably higher than that in the control group. By contrast, the expression of FOXM, C-Jun, and AP1 was reversed after NAC treatment relative to that in the DM group [Figure 1i-l]. These findings indicate that NAC treatment ameliorated the degree of bone destruction in mice with diabetes periodontitis.
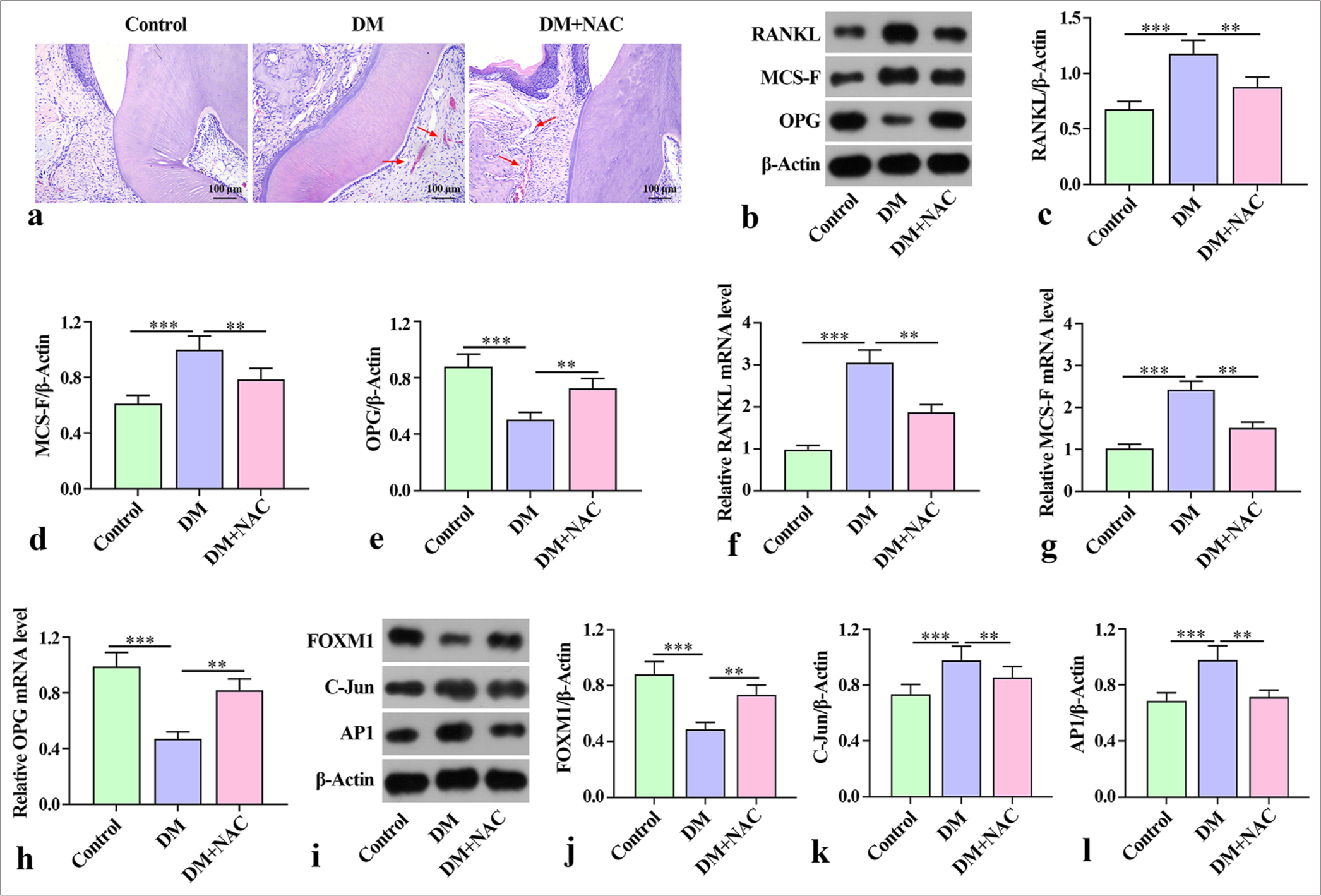
- Effect of ROS inhibition on bone destruction in mice with diabetes periodontitis. (a) Histology of periodontal tissues in different groups of mice obtained via H&E staining. (b-e) Expression of bone resorption-related factors RANKL, MCS-F, and OPG in the serum of mice examined by Western blot. (f-h) Expression of bone resorption-related factors RANKL, MCS-F, and OPG mRNA in the serum of mice detected using qRT-PCR. (i-l) Expression of FOXM1, C-Jun, and AP1 in the mandibles of mice analyzed using Western blot. The experiment was repeated 3 times. ROS: Reactive oxygen species, H&E: hematoxylin–eosin, RANKL: Receptor activator for nuclear factor κB ligand, MCS-F: Macrophage colony-stimulating factor, OPG: Osteoprotegerin, qRT-PCR: Quantitative reverse transcription polymerase chain reaction, FOXM1: Forkhead box protein M1, AP1: Activator protein-1, DM: Diabetes periodontitis model, NAC: ROS inhibitor N-acetylcysteine. ✶✶P < 0.01, ✶✶✶P < 0.001.
ROS inhibition attenuated the inflammatory response in mice with diabetes periodontitis
The qRT-PCR results showed a substantial increase in the mouse jaw levels of inflammatory factors IL-1β, IL-10, and iNOS in the mice with diabetes periodontitis in the DM group compared with the mice in the control group. Remarkably, the levels of these inflammatory factors were reduced by NAC treatment [Figure 2a-c]. In the control group, the levels of IL-1β, IL-10, and iNOS protein were detected. Notable increments in IL-1β, iNOS, and IL-10 protein were observed in the DM group, but they were substantially reduced by NAC treatment [Figure 2d-g]. Overall, these findings indicate that NAC treatment played a role in alleviating the inflammatory response in mice with diabetes periodontitis.
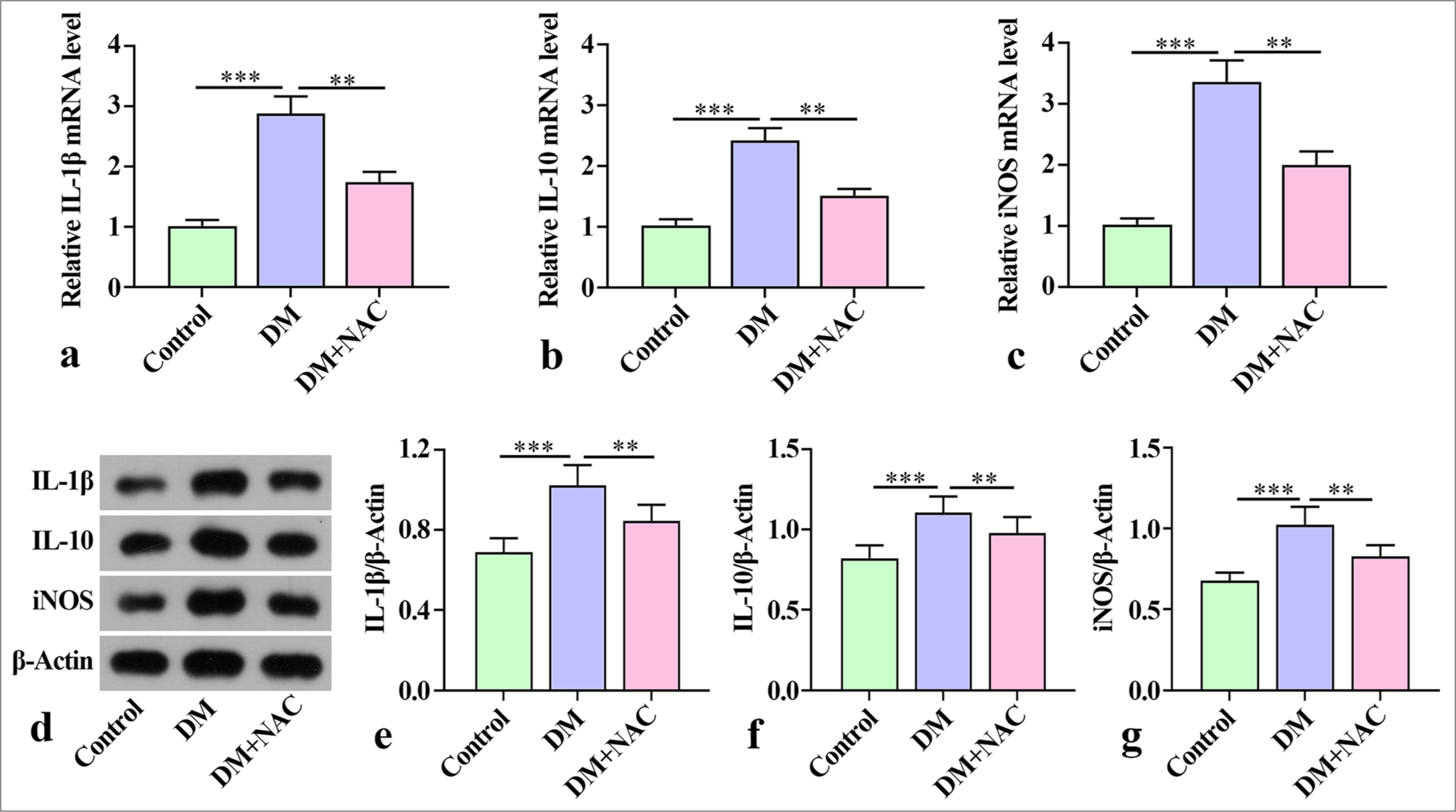
- Effect of ROS inhibition on inflammatory factors in mice with diabetes periodontitis. (a-c) Inflammatory cytokines IL-1β, iNOS, and IL-10 mRNA in the mandibles of mice evaluated by qRT-PCR. (d-g) Levels of inflammatory factors IL-1β, IL-10, and iNOS proteins in mouse mandibles detected by Western blot. The experiment was repeated 3 times. ROS: Reactive oxygen species, IL: Interleukin, iNOS: Inducible nitric oxide synthetase, qRT-PCR: Quantitative reverse transcription polymerase chain reaction, DM: diabetes periodontitis model, NAC: ROS inhibitor N-acetylcysteine. ✶✶P < 0.01, ✶✶✶P < 0.001.
ROS inhibition attenuated oxidative stress in mice with diabetes periodontitis
To further analyze the effect of NAC on oxidative stress in mice with diabetes periodontitis, we examined the levels of MDA, GSH, SOD, and CAT in mouse mandibles. ELISA analysis revealed that the MDA level of mice with diabetes periodontitis considerably increased relative to that in the control group, and GSH, SOD, and CAT were substantially reduced compared with that in control group [Figure 3a-d]. In contrast with the DM group, the level of MDA in tissue decreased remarkably after NAC treatment, but the levels of GSH, SOD, and CAT were notably elevated [Figure 3a-d].
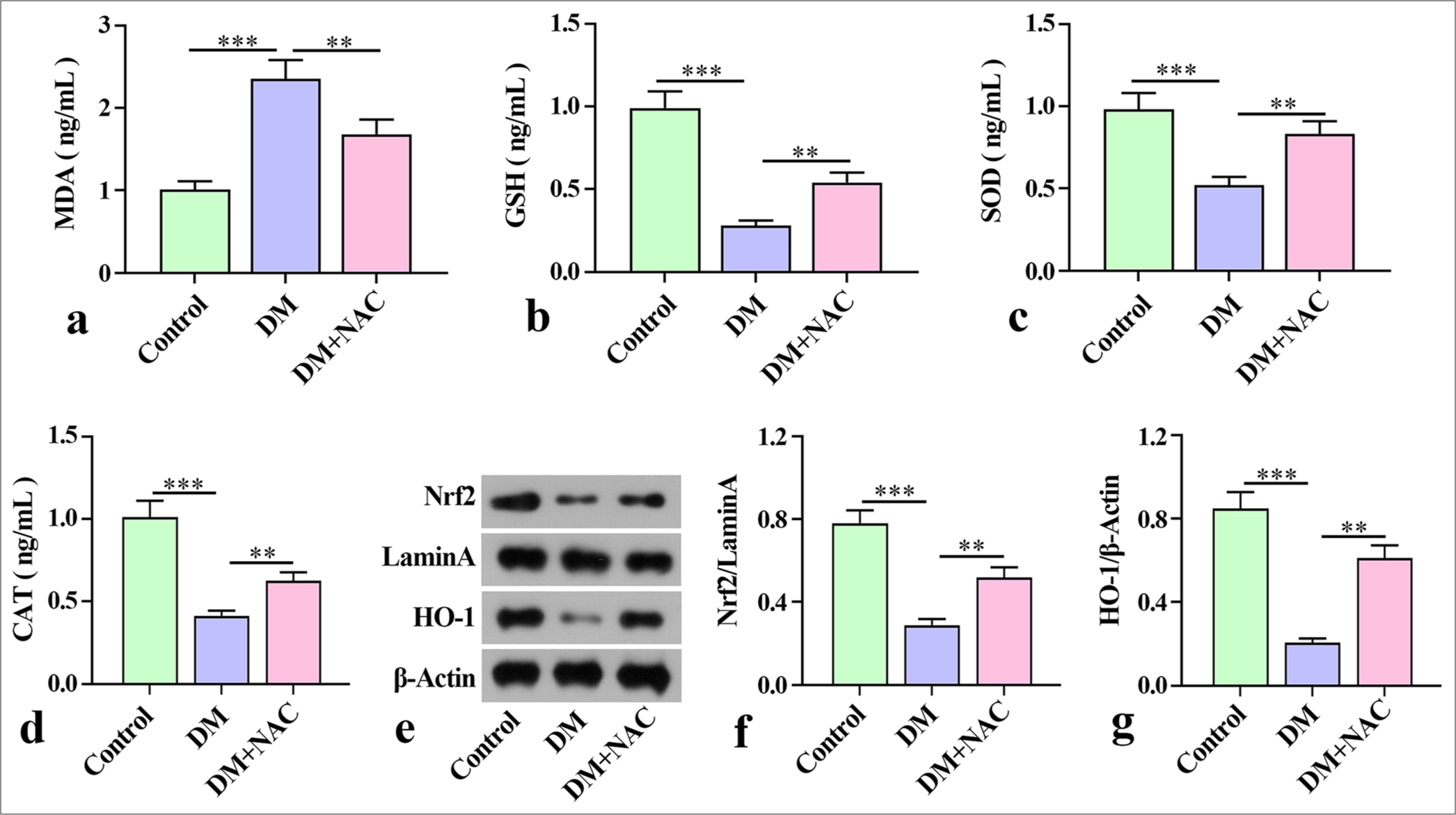
- Effect of the ROS inhibition on oxidative stress in mice with diabetes periodontitis. (a-d) Levels of MDA, SOD, CAT, and GSH in mice mandibles detected by ELISA. (e-g) Expression of proteins Nrf2 and HO-1 examined by Western blot. The experiment was repeated 3 times. ROS: Reactive oxygen species, MDA: Malondialdehyde, SOD: Superoxide dismutase, CAT: Catalase, GSH: Glutathione, ELISA: Enzyme-linked immunosorbent assay, Nrf2: Nuclear factor-erythropoietin 2-related factor 2, HO-1: Hemeoxygenase-1, DM: diabetes periodontitis model, NAC: ROS inhibitor N-acetylcysteine. ✶✶P < 0.01, ✶✶✶P < 0.001.
In addition, the Nrf2 and HO-1 proteins in mice with diabetes periodontitis were estimated through Western blot. The protein expression of Nrf2 and HO-1 in the DM group decreased remarkably relative to that in the control group. With NAC intervention, the protein expression of Nrf2 and HO-1 protein increased remarkably [Figure 3e-g]. These results demonstrate that NAC ameliorated oxidative stress in mice with diabetes periodontitis.
Silencing FOXM1 mediated the ability of AP-1 to enhance ROS production after osteoblast-like differentiation of macrophages
This study also validated whether FOXM1 mediates AP-1 to influence the ability for macrophage osteoclast-like differentiation after ROS production. Western blot showed that sh-FOXM1 reduced the levels of FOXM1 but increased the levels of C-Jun and AP-1 in the macrophage cells [Figure 4a-d]. Knockdown of Jun increased the protein expression of FOXM1 and reduced the protein expression of C-Jun and AP-1 compared with the sh-FOXM1-RANKLLPS-NAC + nonsense-siRNA group. Subsequent experiments revealed that sh-FOXM1 increased AP-1 binding activity in the RANKL-LPS-inducted macrophage cells [Figure 4e]. AP-1 binding activity was considerably reduced after JUNsiRNA compared with that in the sh-FOXM1-RANKL-LPSNAC + nonsense-siRNA group. The high AP-1 binding activity indicated that silencing FOXM1 activated AP-1 activity, which is related to the promotion of diabetes periodontitis. Furthermore, ROS detection showed that the number of positive cells in sh-FOXM1 increased compared with that in the RANKL-LPS group. AP-1 binding activity and ROS production were substantially reduced after NAC treatment compared with those in the sh-FOXM1-RANKLLPS group. The group of cells treated with JUN-siRNA had a smaller number of positive cells compared with the group containing cells treated with nonsense-siRNA. This outcome suggests that silencing FOXM1 increased ROS production when the cells were in an inflammatory state [Figure 4f and g]. Overall, these findings indicate that sh-FOXM1 mediated the ability of AP-1 to enhance ROS production after osteoblast-like differentiation of macrophages.
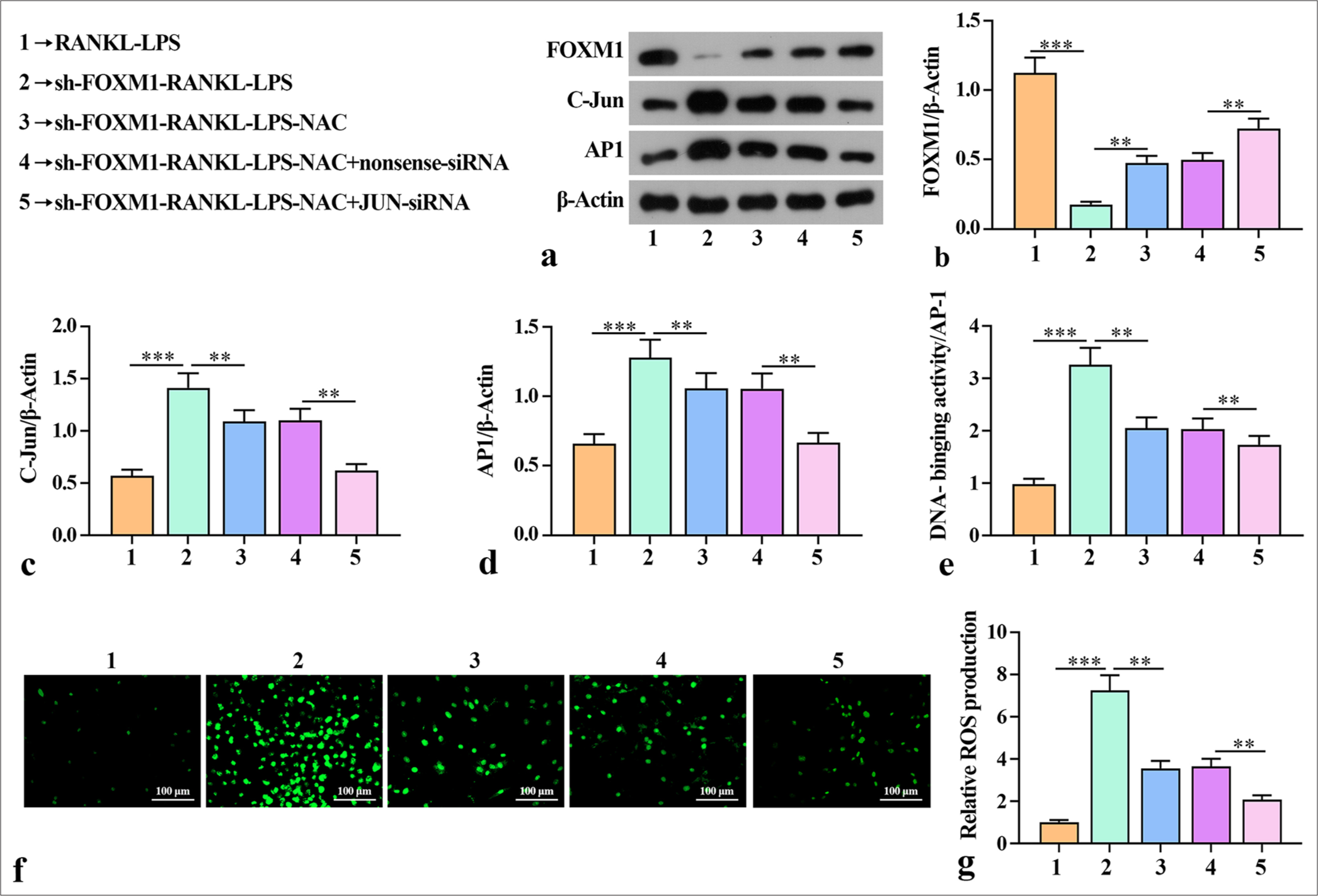
- Silencing of FOXM1 mediated the effects of AP-1 on ROS generation during macrophage osteoclast-like differentiation. (a-d) Levels of FOXM1, C-Jun, and AP1 in macrophage cells determined by Western blot. (e) EMSA analysis of AP-1 binding activity in macrophage cells. (f and g) Levels of ROS in macrophage cells determined by the DCFH-DA probe. The experiment was repeated 3 times. RANKL: Receptor activator for nuclear factor κ B ligand, LPS: Lipopolysaccharide, FOXM1: Forkhead box protein M1, NAC: ROS inhibitor N-acetylcysteine, JUN: JUN corresponding to the core subunit C-Jun in AP1 heterodimers, ROS: Reactive oxygen species, AP-1: Activator protein-1, EMSA: Electrophoretic mobility shift assay, DCFH-DA: Dichlorodihydrofluorescein diacetate. ✶✶P < 0.01, ✶✶✶P < 0.001.
Silencing of FOXM1-regulated ROS production mediated AP-1 activation to promote macrophage osteoclast differentiation
Osteoclast differentiation is controlled by the activation of multiple genes that respond when RANKL binds to RANK receptors. TRAP, OSCAR, and NFATc1 play a critical role in the differentiation of osteoclasts.[21] We studied how FOXM1 affected the expression of TRAP, OSCAR, and NFATc1 induced by RANKL-LPS. Western blot was implemented to verify the effects of FOXM1 on TRAP, OSCAR, and NFATc1 protein expression. TRAP, OSCAR, and FATc1 protein levels were promoted after sh-FOXM1-RANKL-LPS treatment, but TRAP, OSCAR, and NFATc1 expression was notably inhibited by sh-FOXM1-RANKL-LPS-NAC [Figure 5a-d]. Compared with the protein expression of TRAP, OSCAR, and NFATc1 in the sh-FOXM1-RANKL-LPS-NAC+nonsense-siRNA group, the protein expression in the sh-FOXM1-RANKLLPS-NAC+JUN-siRNA group decreased considerably. In addition, the mRNA levels of TRAP, OSCAR, and NFATc1 in the cell lysates were assessed by qRT-PCR. The results showed that TRAP, OSCAR, and NFATc1 mRNA expression was notably promoted by sh-FOXM1 but substantially inhibited by sh-FOXM1-RANKL-LPS-NAC [Figure 5e-g].
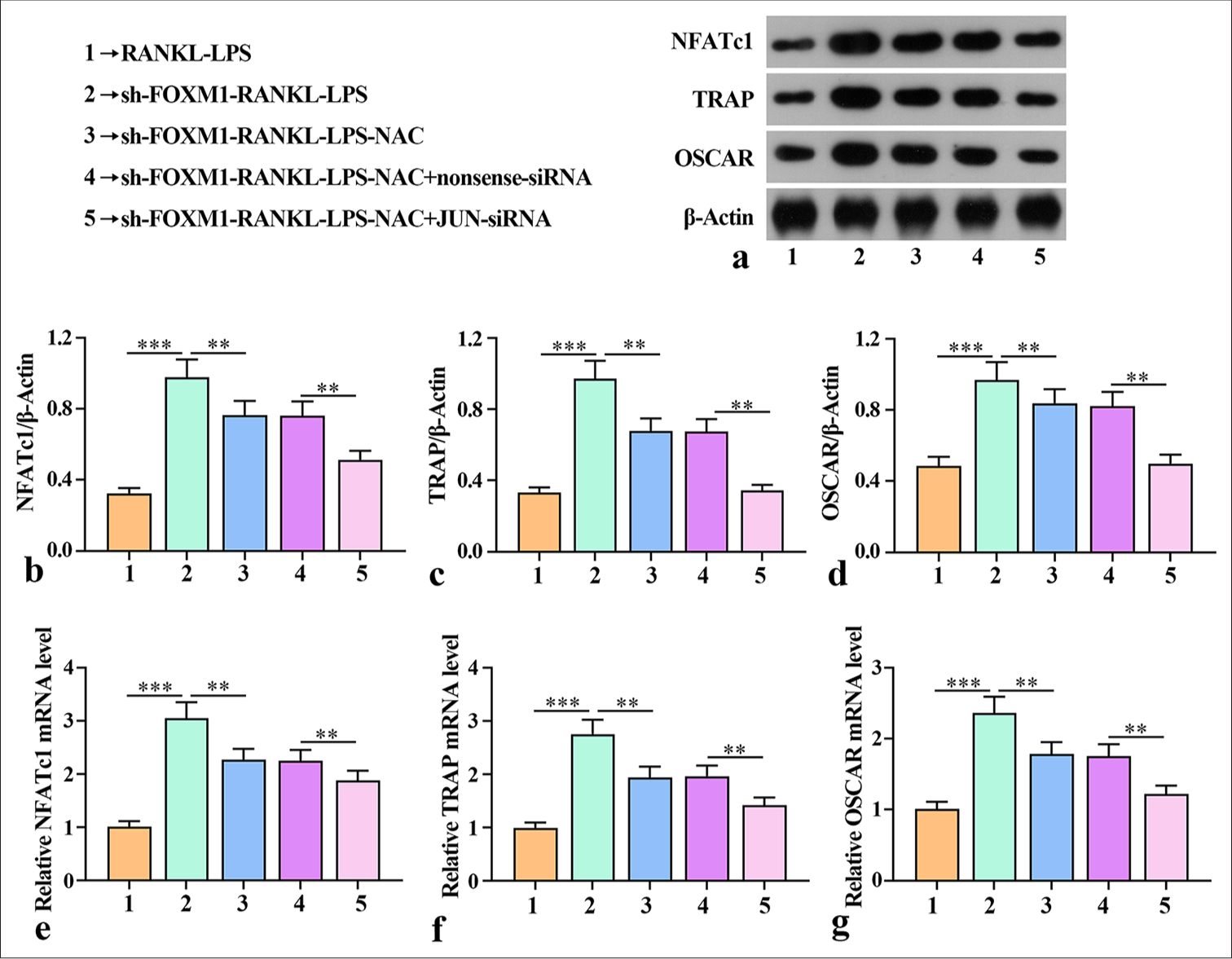
- Silencing of FOXM1-regulated ROS production mediated AP-1 activation in the osteoclast differentiation of macrophages. (a-d) Expression of NFATc1, TRAP, and OSCAR proteins in cell lysates evaluated by Western blot. (e-g) Levels of NFATc1, TRAP, and OSCAR mRNA in cell lysates assessed by qRT-PCR. The experiment was repeated 3 times. RANKL: Receptor activator for nuclear factor κ B ligand, LPS: lipopolysaccharide, FOXM1: Forkhead box protein M1, NAC: ROS inhibitor N-acetylcysteine, JUN: JUN corresponding to the core subunit C-Jun in AP1 heterodimers, ROS: Reactive oxygen species, AP-1: Activator protein-1, NFATc1: Nuclear factor-activated T cell c1, TRAP: Tartrate-resistant acid phosphatase, OSCAR: Osteoclast-associated receptor, qRT-PCR: Quantitative reverse transcription polymerase chain reaction. ✶✶P < 0.01, ✶✶✶P < 0.001.
The mRNA expression levels of TRAP, OSCAR, and NFATc1 in the sh-FOXM1-RANKL-LPS-NAC + JUN-siRNA group were much lower than those in the sh-FOXM1-RANKL-LPSNAC + nonsense-siRNA group. These results demonstrate that silencing of FOXM1-regulated ROS production mediated AP-1 activation to promote macrophage osteoclast differentiation.
DISCUSSION
Diabetes and periodontal disease, two common and highly prevalent diseases, affect human health. Periodontal disease is the sixth major complication of diabetes, and the incidence of periodontal disease in diabetic patients is considerably increased and manifests as inflammation and aggravated periodontal destruction.[22] Therefore, exploring strategies to address diabetes-related periodontal disease is particularly important.[5] FOXM1 is a transcription factor that promotes cell proliferation and serves various functions in vivo; it plays roles in cell proliferation, differentiation, migration, DNA repair, surfactant synthesis, and establishment of cellular junctions.[23,24] Its mechanisms of action in diabetes and periodontal disease remain unclear and require further study. This study explored the effect of ROS production on diabetes-related periodontal disease by establishing a mouse model of diabetes-related periodontal disease. The results indicated that ROS inhibition alleviated bone destruction, inflammatory response, and oxidative stress levels in mice with diabetes-related periodontal disease. Further assessment was conducted on the effect of FOXM1 on ROS and its role in diabetes periodontitis. The results revealed that FOXM1 silencing regulated ROS production and thus mediated AP-1 activation, resulting in the exacerbation of the inflammatory response in diabetes periodontitis.
ROS plays an important role in periodontitis, promoting its progression and exacerbating the inflammatory response.[19] NAC, a precursor of glutathione, is a sulfhydryl-containing thiol antioxidant that has antioxidant, anti-inflammatory, and ROS-scavenging properties; it prevents bone loss and increases osteoblast activity in diabetic rats.[25,26] To further clarify the role of ROS in mice with diabetes periodontitis, we treated mice with the ROS inhibitor NAC. The results showed that NAC intervention alleviated epithelial defects and rupture in periodontal tissues, reduced inflammatory cell infiltration, decreased the mRNA and protein expression of bone-resorbing factors RANKL and MCS-F, and increased OPG. Notably, with NAC intervention, the protein expression of FOXM1 substantially increased, whereas that of C-Jun and AP1 decreased remarkably, indicating that NAC alleviated the degree of bone destruction in the mice with diabetes periodontitis. These results are consistent with the finding that NAC reduces alveolar bone loss in cases of experimental periodontitis in streptozotocin-induced diabetic rats.[26]
The secretion of IL-1β, IL-10, and iNOS is essential for the progression of diabetes periodontitis.[27,28] These cytokines have been found to be involved in bone loss and the inflammatory response of bone marrow mesenchymal stem cells (BMMSCs).[29,30] Our results showed an increase in the levels of IL-4, IL-13, and IL-5 in the jawbone of mice with diabetes periodontitis. NAC treatment inhibited the release of inflammatory markers IL-1β, IL-6, and iNOS. Moreover, this study found a substantial reduction in tissue MDA levels after NAC treatment and remarkable increments in the levels of GSH, SOD, and CAT. NAC mitigated oxidative stress in the mice with diabetes periodontitis. Similar results have been obtained in previous studies.[20,31,32] Nrf2 functions as a primary regulator of the expression of various antioxidants.[33] HO-1 is a downstream gene of Nrf2 and under the control of Nrf2. The consistency between the in vivo and in vitro results of Nrf2 and HO-1 strongly suggest their synergy, further indicating that melatonin-derived carbon dots may activate the Nrf2/HO-1 pathway to enhance their antioxidant capacity, thereby exerting anti-inflammatory effects and protecting against periodontal damage.[34] Furthermore, a study showed that NAC increases the protein and mRNA expression of siNrf2 in osteogenic differentiation of periodontal ligament stem cells under cyclic mechanical stretch.[35] In the present study, a substantial increase in the protein expression of Nrf2 and HO-1 was observed after NAC intervention, and this increment ameliorated oxidative stress in the mice with diabetes periodontitis.
FOXM1 is widely recognized for its essential roles in regulating a range of cellular activities.[36] Interfering with FOXM1 gene expression enhances the osteogenic differentiation capacity of BMMSCs.[37] Upregulation of FOXM1 enhances the resistance of BMMSCs to LPS-induced apoptosis in alveolar epithelial cells.[38] Moreover, suppressing FOXM1 expression can promote osteoclast differentiation and RANKL/OPG in HPDLCs.[17] These findings indicate that the function of FOXM1 varies across different cell types. Meanwhile, ROS production can modulate different redox-sensitive transcription factors, such as AP-1, through the transcription factor FOXM1 to counteract oxidative damage.[39-41] The crucial role of AP-1 in osteoblast differentiation and function has been established in the previous studies.[42] ROS production is a key factor in AP-1 activation, which leads to periodontal inflammation.[14,43] However, whether FOXM1-regulated ROS production mediates the activation of AP-1 or not and FOXM1’s effect on macrophage osteoclast differentiation is unclear. In this study, silencing FOXM1 increased the binding activity of AP-1 and enhanced the capacity for ROS production. Conversely, knockdown of JUN corresponding to the core subunit C-Jun in the macrophage AP1 heterodimers reduced AP-1 binding activity and ROS production. In addition, sh-FOXM1 increased the mRNA and protein levels of osteoclast-related factors NFATc1, TRAP, and OSCAR, whereas JUN-siRNA decreased their expression. These results indicate that silencing FOXM1-regulated ROS production mediated AP-1 activation and promoted macrophage osteoclast differentiation. The synergistic effects of these molecular-level changes (gene expression and protein level alterations) influenced the differentiation of macrophages into osteoclasts and altered bone resorption in mice. Therefore, the molecular mechanism of silencing FOXM1 promotes the inflammatory response and pathological changes in diabetes periodontitis. FOXM1 is present in mouse and human diseases and plays an important role. The results of this study enhance the understanding of the role of FOXM1 in clinical diabetes periodontitis and provide new theoretical support for the clinical prevention and treatment of human diabetes periodontitis. However, the specific roles and mechanisms of FOXM1/ROS/AP-1 in diabetes periodontitis and the etiology and treatment of diabetes periodontitis still require further exploration.
SUMMARY
In this study, ROS inhibition alleviated bone damage, reduced the inflammatory response, and diminished oxidative stress in mice with diabetes periodontitis. In the future, we will confirm the efficacy of FOXM1 in alleviating clinical diabetes periodontitis using alternative experimental techniques.
Our findings provide new targets for alleviating diabetes periodontitis, particularly FOXM1, and offer new strategies and a theoretical foundation for the clinical prevention and treatment of diabetes periodontitis in humans.
AVAILABILITY OF DATA AND MATERIALS
The data that support the findings of this study are available from the corresponding author on reasonable request.
ABBREVIATION
FOXM1: Forkhead box protein M1
AP-1: Activator protein-1
ROS: Reactive oxygen species
NAC: N-Acetylcysteine
DM: Diabetic periodontitis model
RANKL: Receptor activator for nuclear factor κ B Ligand
LPS: Lipopolysaccharide
ShRNA: Short Hairpin RNA
ELISA: Enzyme-linked immunosorbent assay
EMSA: Electrophoretic mobility shift assay
OPG: Osteoprotegerin
MCS-F: Macrophage colony-stimulating factor
IL: Interleukin
iNOS: Inducible nitric oxide synthetase
NFATc1: Nuclear factor-activated T-cells c1
TRAP: Tartrate resistant acid phosphatase
OSCAR: Osteoclast-associated receptor
A: Adenosine
C: Cytosine
G: Guanine
T: Thymine
AUTHOR CONTRIBUTIONS
YY and DPR: Designed the research study and wrote the first draft; BBP and JLH: Performed the research; BXY: Analyzed the data. All authors contributed to editorial changes in the manuscript. All authors read and approved the final manuscript. All authors have participated sufficiently in the work and agreed to be accountable for all aspects of the work.
ETHICS APPROVAL AND CONSENT TO PARTICIPATE
All experimental protocols of this study were approved by West China Hospital of Stomatology Sichuan University ethics committee (No: WCHSIRB-D-2022-418). Informed consent is not required as there are no patients in this article.
CONFLICT OF INTEREST
The authors declare no conflict of interest.
EDITORIAL/PEER REVIEW
To ensure the integrity and highest quality of CytoJournal publications, the review process of this manuscript was conducted under a double-blind model (authors are blinded for reviewers and vice versa) through an automatic online system.
FUNDING
This study is supported by Sichuan Provincial Natural Science Foundation (2023NSFSC1515).
References
- Update on the bidirectional link between diabetes and periodontitis. Adv Exp Med Biol. 2022;1373:231-40.
- [CrossRef] [PubMed] [Google Scholar]
- Genetics of diabetes mellitus and diabetes complications. Nat Rev Nephrol. 2020;16:377-90.
- [CrossRef] [PubMed] [Google Scholar]
- Periodontitis in US adults: National health and nutrition examination survey 2009-2014. J Am Dent Assoc. 2018;149:576-588.e6.
- [CrossRef] [PubMed] [Google Scholar]
- Vitamin D attenuates human gingival fibroblast inflammatory cytokine production following advanced glycation end product interaction with receptors for AGE. J Periodontal Res. 2019;54:154-63.
- [CrossRef] [PubMed] [Google Scholar]
- Diabetes as a potential risk for periodontitis: Association studies. Periodontol 2020. 2000;83:40-5.
- [CrossRef] [PubMed] [Google Scholar]
- Influence of periodontal disease on changes of glycated hemoglobin levels in patients with type 2 diabetes mellitus: A retrospective cohort study. J Periodontol. 2017;88:17-25.
- [CrossRef] [PubMed] [Google Scholar]
- Reactive oxygen species and human inflammatory periodontal diseases. Biochemistry (Mosc). 2005;70:619-28.
- [CrossRef] [PubMed] [Google Scholar]
- Peroxidative stress and nutrient antioxidant status in periodontal disease. Int J Biol Med Res. 2013;4:3189-3191.
- [Google Scholar]
- Role of reactive oxygen species in cancer progression. Curr Pharmacol Rep. 2019;5:79-86.
- [CrossRef] [Google Scholar]
- Genetic aspects of inflammation and cancer. Biochem J. 2008;410:225-35.
- [CrossRef] [PubMed] [Google Scholar]
- The Mbd4 DNA glycosylase protects mice from inflammation-driven colon cancer and tissue injury. Oncotarget. 2016;7:28624-36.
- [CrossRef] [PubMed] [Google Scholar]
- Clinical implication of perioperative inflammatory cytokine alteration. Acta Anaesthesiol Taiwan. 2015;53:23-8.
- [CrossRef] [PubMed] [Google Scholar]
- Role of AP-1 transcriptional factor in development of oxidative and nitrosative stress in periodontal tissues during systemic inflammatory response. Ukr Biochem J. 2019;91:80-5.
- [CrossRef] [Google Scholar]
- Role of reactive oxygen species and antioxidants in periodontal disease. J Cell Biotechnol. 2021;7:125-40.
- [CrossRef] [Google Scholar]
- Force-induced decline of FOXM1 in human periodontal ligament cells contributes to osteoclast differentiation. Angle Orthod. 2019;89:804-11.
- [CrossRef] [PubMed] [Google Scholar]
- FOXM1 promotes allergen-induced goblet cell metaplasia and pulmonary inflammation. Mol Cell Biol. 2013;33:371-86.
- [CrossRef] [PubMed] [Google Scholar]
- Reactive oxygen species in periodontitis. J Indian Soc Periodontol. 2013;17:411-6.
- [CrossRef] [PubMed] [Google Scholar]
- The effect of N-acetylcysteine on periodontitis: A mini-review of literature. Res Soc Development. 2021;10:e42610515134.
- [CrossRef] [Google Scholar]
- Aspirin inhibits RANKL-induced osteoclast differentiation in dendritic cells by suppressing NF-κB and NFATc1 activation. Stem Cell Res Ther. 2019;10:375.
- [CrossRef] [PubMed] [Google Scholar]
- Dysregulated FOXM1 signaling in the regulation of cancer stem cells. Semin Cancer Biol. 2022;86:107-21.
- [CrossRef] [PubMed] [Google Scholar]
- Targeting forkhead box M1 transcription factor in breast cancer. Biochem Pharmacol. 2018;154:407-13.
- [CrossRef] [PubMed] [Google Scholar]
- N-acetylcysteine regulates dental follicle stem cell osteogenesis and alveolar bone repair via ROS scavenging. Stem Cell Res Ther. 2022;13:466.
- [CrossRef] [PubMed] [Google Scholar]
- N-acetylcysteine decreases alveolar bone loss on experimental periodontitis in streptozotocin-induced diabetic rats. J Periodontal Res. 2012;47:793-9.
- [CrossRef] [PubMed] [Google Scholar]
- Redox status in periodontal and systemic inflammatory conditions including associated neoplasias: Antioxidants as adjunctive therapy? Infect Disord Drug Targets. 2009;9:415-27.
- [CrossRef] [PubMed] [Google Scholar]
- The effect of melatonin on bone loss, diabetic control, and apoptosis in rats with diabetes with ligature-induced periodontitis. J Periodontol. 2016;87:e35-43.
- [CrossRef] [PubMed] [Google Scholar]
- Immunomodulation in the treatment of periodontitis: Progress and perspectives. Front Immunol. 2021;12:781378.
- [CrossRef] [PubMed] [Google Scholar]
- Nacetyl cysteine inhibits the lipopolysaccharideinduced inflammatory response in bone marrow mesenchymal stem cells by suppressing the TXNIP/NLRP3/IL1β signaling pathway. Mol Med Rep. 2020;22:3299-306.
- [CrossRef] [Google Scholar]
- Beneficial effects of N-acetylcysteine on hepatic oxidative stress in streptozotocin-induced diabetic rats. Canadian Journal of Physiology and Pharmacology. 2018;96:412-418.
- [CrossRef] [PubMed] [Google Scholar]
- N-acetylcysteine supplementation did not reverse mitochondrial oxidative stress, apoptosis, and inflammation in the salivary glands of hyperglycemic rats. Nutr Diabetes. 2021;11:35.
- [CrossRef] [PubMed] [Google Scholar]
- Nrf2: Redox and metabolic regulator of stem cell state and function. Trends Mol Med. 2020;26:185-200.
- [CrossRef] [PubMed] [Google Scholar]
- Melatonin-derived carbon dots with free radical scavenging property for effective periodontitis treatment via the Nrf2/HO-1 pathway. ACS Nano. 2024;18:8307-24.
- [CrossRef] [PubMed] [Google Scholar]
- Nrf2 activation is involved in osteogenic differentiation of periodontal ligament stem cells under cyclic mechanical stretch. Exp Cell Res. 2021;403:112598.
- [CrossRef] [PubMed] [Google Scholar]
- FOXO transcription factors at the interface of metabolism and cancer. Int J Cancer. 2017;141:2379-91.
- [CrossRef] [PubMed] [Google Scholar]
- Knockdown of foxM1 strengthens osteogenic differentiation ability of bone marrow mesenchymal stem cells. Chin J Tissue Eng Res. 2019;23:673.
- [Google Scholar]
- Over-expression of BMSC foxM1 attenuates LPS-induced apoptosis of alveolar epithelial cells China: Sun Yat-sen University of Medical Sciences; 2019. p. :833-41.
- [Google Scholar]
- Activation of AKT/AP1/FoxM1 signaling confers sorafenib resistance to liver cancer cells. Oncol Rep. 2019;42:785-96.
- [CrossRef] [PubMed] [Google Scholar]
- APE1/Ref-1 as an emerging therapeutic target for various human diseases: Phytochemical modulation of its functions. Exp Mol Med. 2014;46:e106.
- [CrossRef] [PubMed] [Google Scholar]
- FOXM1 in Cancer: Interactions and vulnerabilities. Cancer Res. 2017;77:3135-9.
- [CrossRef] [PubMed] [Google Scholar]
- Photodynamic activation as a molecular switch to promote osteoblast cell differentiation via AP-1 activation. Sci Rep. 2015;5:13114.
- [CrossRef] [PubMed] [Google Scholar]
- Reversine inhibits MMP-3, IL-6 and IL-8 expression through suppression of ROS and JNK/AP-1 activation in interleukin-1β-stimulated human gingival fibroblasts. Arch Oral Biol. 2019;108:104530.
- [CrossRef] [PubMed] [Google Scholar]