Translate this page into:
Astragalus polysaccharide attenuates retinal ischemia reperfusion-induced microglial activation through sortilin-related vacuolar protein sorting 10 domain containing receptor 2/laminin subunit alpha 1 upregulation
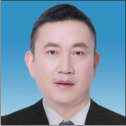
*Corresponding author: Zhikun Zheng, Department of Ophthalmologic, The Affiliated Hospital of Yunnan University (Second People’s Hospital of Yunnan Province, Yunnan Eye Hospital), Kunming, Yunnan, China. drzhengzhikun@163.com
-
Received: ,
Accepted: ,
How to cite this article: Li J, Li H, Wei C, Chen C, Zheng Z. Astragalus polysaccharide attenuates retinal ischemia reperfusion-induced microglial activation through sortilin-related vacuolar protein sorting 10 domain containing receptor 2/laminin subunit alpha 1 upregulation. CytoJournal. 2025;22:2. doi: 10.25259/Cytojournal_131_2024
Abstract
Objective:
Microglial activation is a hallmark of pathogenic retinal conditions such as retinal ischemia-reperfusion (RIR). While sortilin-related vacuolar protein sorting 10 domain containing receptor 2 (Sorcs2) and laminin subunit alpha 1 (Lama1) have been implicated in neuroinflammatory processes, their roles in regulating microglial activation in RIR are not reported. The current work studied the potential of Sorcs2 and Lama1 as negative regulators of microglial activation in RIR and assessed the therapeutic potential of Astragalus polysaccharide (AP).
Material and Methods:
Transcriptome profiling was conducted in retinal specimens of RIR group 72 h after RIR induction. Oxygen-glucose deprivation/reperfusion (OGD/R) in rat microglial cells was employed as the cellular induction model of RIR. The functional role of Sorcs2 and Lama1 in dictating microglial activation was investigated in vitro and in vivo using lentivirus-based gene expression. Further, the potential effect of AP on RIR-mediated microglial activation was investigated.
Results:
Sorcs2 and Lama1 were identified as two downregulated genes in retinal samples following RIR. OGD/R induction triggered pro-inflammatory microglial activation and induced the downregulation of Sorcs2 and Lama1. Sorcs2 or Lama1 overexpression hindered OGD/R-induced microglial activation in vitro and attenuated inflammatory expansion of microglia cells in RIR-induced rat retinal samples. AP treatment was able to neutralize the oxidative stress, promote the expression of Sorcs2 and Lama1, and suppress microglial activation.
Conclusion:
Our findings pinpoint Sorcs2 and Lama1 as negative regulators of microglial activation in RIR. AP could be employed as an antioxidant to attenuate microglial activation and ameliorate the inflammatory damages in RIR.
Keywords
Retinal ischemia
Reperfusion injury
Glucose
Laminin
Astragalus
INTRODUCTION
Ischemic retinal disorders arise from the interruption of retinal blood supply followed by reperfusion, leading to a cascade of pathological events, including neurodegeneration, inflammatory damage, and alterations in retinal microvasculature.[1,2] The elevation of intraocular pressure could induce ischemia and hypoxia in retinal tissues, with subsequent cell death of retinal ganglion cells (RGCs) causing vision loss.[3] Blood reperfusion after ischemia also triggers detrimental physical and physiological consequences in retinal tissues. Concurrently, retinal ischemia-reperfusion (RIR) can also induce a burst of reactive oxygen species (ROS) and oxidative damage within the retinal endothelial layer.[4,5] The influx of immune cells and production of inflammatory mediators can trigger a secondary inflammatory cascade, exacerbating retinal damage.[6,7] Therefore, targeting inflammatory and oxidative injuries has been proposed as the main strategy to alleviate RIR-induced retinal damage.[8]
To elucidate the pathological mechanisms underlying RIR, researchers have developed a pressure-induced retinal ischemia model in rats. This model involves cannulation of the anterior chamber to elevate intraocular pressure, followed by reperfusion upon pressure normalization.[9] The animal model allows the characterization of neuronal apoptosis, vascular permeability, and immune cell profile alteration in retinal tissues after RIR induction.[10-13] For example, it has been demonstrated that degeneration initially occurs in neurons within the RGC layer post-RIR, followed by neuronal death in both the inner core layer and the photoreceptors.[10,11] Furthermore, RIR may lead to the compromise of the capillary vascular structure in the retina, including heightened capillary permeability.[13]
In the retina and central nervous system, microglia serve as the resident macrophages to uphold tissue homeostasis.[14] Within a normal retina, microglial cells are mainly located within the inner plexiform sites and ganglion cell layer, actively participating in the monitoring of neuronal tissues by carrying out phagocytosis.[15] Under pathogenic conditions such as RIR, the over-activation of microglia and the change of functional phenotype exacerbate the disease progression.[16] Unusual stimulation of microglia promotes the production of inflammatory mediators, which then attract circulating macrophages to trigger long-lasting immune reactions in the retina.[17-19] Thus, microglial activation aggravates retinal neurodegeneration and has been recognized as a hallmark of pathogenic retinal conditions such as RIR.[20-22] Furthermore, evidence suggests that reshaping the phenotype of microglial cells could increase the susceptibility of retinal tissues to age-related macular degeneration.[23] However, candidate genes implicated in the microglial activation have not been comprehensively characterized.
In our current analysis, the transcriptome of retinal tissues was profiled 72 h post-RIR induction using a rat model. Our findings revealed that sortilin-related vacuolar protein sorting 10 domain containing receptor 2 (Sorcs2) and laminin subunit alpha 1 (Lama1) were downregulated in the retinal specimens following the RIR condition. To elucidate the functional roles of Sorcs2 and Lama1 in microglial activation, we also developed a cell model to mimic RIR conditions using microglial cells. In addition, to examine their roles in a rat RIR model, we delivered lentiviruses carrying the expression sequences for Sorcs2 and Lama1. Furthermore, we evaluated the potential impact of an antioxidant, Astragalus polysaccharide (AP), on RIR-induced microglial activation in vitro and in vivo.
MATERIAL AND METHODS
Cell culture and treatment
Highly aggressively proliferating immortalized (HAPI) cells (rat microglia cells, item number HC-R037, Binsui Biotech, Shanghai, China) were acquired. The cells were tested for mycoplasma contamination and were subjected to short tandem repeat profiling for authentication by the supplier. They were cultivated using Dulbecco’s modified eagle medium (DMEM) (catalog number PM150210, lot number 20230305, Procell, Wuhan, Hubei, China) containing 10% fetal bovine serum (catalog number 164210-500, lot number A2324-500, Procell, Wuhan, Hubei, China) and 1% penicillin-streptomycin (catalog number PB180120, lot number 20230228, Procell, Wuhan, Hubei, China) in a humidified incubator containing 5% carbon dioxide (CO2) and 95% air, at 37°C. An RIR cell model was created using oxygen-glucose deprivation/reperfusion (OGD/R) conditions in vitro. Control cells were cultivated at 37°C in 5% CO2 and 95% air for 8 h using DMEM containing 4.5 g/L D-glucose (catalog number PM150210, lot number 20230305, Procell, Wuhan, Hubei, China). Experimental cells underwent ischemia in an anaerobic chamber with glucose-free DMEM (catalog number PM150275, lot number 20230209, Procell, Wuhan, Hubei, China) for 8 h. Reperfusion involved returning cells to standard conditions with complete DMEM (4.5 g/L D-glucose) for 24 h. APs (catalog number HY-159066, lot number 220415, MedChemExpress, Shanghai, China) were used at 1–100 μg/mL for 48 h.
Animal model
Male Sprague–Dawley rats (8-week-old, 200–225 g) were purchased from Weitong Lihua Experimental Animal Technology Co., Ltd. (Beijing, China). The retinal RIR model was established as previously reported. Tropicamide Ophthalmic Solution (lot number 230617, Santen Pharmaceutical Co., Ltd., Osaka, Japan) solution was used to dilate pupils after anesthesia with intraperitoneal injection of sodium pentobarbital (50 mg/kg body weight). Ischemia was induced by inserting a needle into the anterior chamber, connected to a saline reservoir 1088 mm above the eye. Fundus pallor confirmed increased intraocular pressure. After 60 min, reperfusion was initiated by lowering the reservoir. Controls underwent the same procedure without a pressure increase. Erythromycin ointment (catalog number 1303791, lot number 221207, Valeant Pharmaceuticals International, Laval, Quebec, Canada) was applied to prevent potential infection. Animals were maintained in a standard animal housing room under controlled environmental conditions (22 ± 2°C and a 12-h light/dark cycle). Environmental enrichment was provided to promote natural behaviors. Daily health checks were performed by trained personnel to monitor for signs of illness, injury, or distress. Body weight and food intake were recorded weekly. Any animals showing signs of poor health or significant weight loss (>20% of body weight) were excluded from the study and humanely euthanized by CO2 asphyxiation (with a flow rate of 20% chamber volume per minute) until breathing ceased, followed by cervical dislocation to ensure death.
Lentiviral treatment: lentivirus (1 × 109 plaque-forming unit) was injected through the tail vein 3-day pre-RIR. AP treatment: 5 mg/kg/day intraperitoneally. Each group had five animals. Three days post-RIR, animals were euthanized by CO2 asphyxiation (with a flow rate of 20% chamber volume per minute) until breathing ceased, followed by cervical dislocation to ensure death. Retinal tissues were harvested from terminally dead mice. The experimental study involving animals was approved by the institutional ethics committee for animal usage, and all procedures were conducted in compliance with institutional guidance for the humane treatment of laboratory animals and Institutional Animal Care and Use Committee guidelines of the Laboratory Animal Center of Peking University.
Transcriptome analysis
Sample Collection and RNA Extraction: Retinal tissues from control and RIR groups were harvested 72-h post-RIR induction. The total RNA sample was purified using a TRIzol-based isolation kit (catalog number R401-01, lot number 20221015, Vazyme, Nanjing, Jiangsu, China).
RNA-Sequencing analysis: RNA-seq libraries were generated using the Kapa Chain Directional mRNA library preparation kit (catalog number KK8420, lot number KD2022, Kapa Biosystems, Tokyo, Japan). High-throughput sequencing was conducted on the Illumina HiSeq 2500 platform (Illumina, San Diego, CA, USA). Raw data alignment: Raw sequencing reads (fastq files) were aligned to the rat genome using Hisat2 (version 1.3.2). Differential expression analysis: The R package edgeR was employed to identify differentially expressed genes (DEGs). Significance Criteria: DEGs were defined as genes with |log2(Fold change)| > 1 and adjusted P-value (q-value) < 0.05.
Real-time quantitative polymerase chain reaction (RT-qPCR)
Gene expression analysis was conducted using RT-qPCR. RNA sample was isolated from retinal samples using the RNeasy Mini Kit (catalog number 74104, lot number 163074064, Qiagen, Hilden, Germany). The generation of first-strand complementary DNA was conducted through the PrimeScript™ RT Reagent Kit (catalog number RR037A, lot number AK71340, Takara, Dalian, Liaoning, China). Quantitative polymerase chain reaction (qPCR) was performed with the SYBR Green qPCR Master Mix (catalog number HY-K0501, lot number 220908, MedChemExpress, Shanghai, China). The quantification of relative gene expression was based on the 2-ΔΔCT method, with β-actin being used as the endogenous control for normalization. The following primers are used in the study: Sorcs2 Forward: CGACATCAACAAACCGAACCAAATAC, Sorcs2 Reverse: TTGCCAAGAAGACTCCCTTCACCC, Lama1 Forward: CCCGAAACTGGAAAGTGTCTGAAC, Lama1 Reverse: GGCTGAAACTGAAAGGAGGGTGAT, β-actin Forward: ACATCCGTAAAGACCTCTATGCCAACA, β-actin Reverse: GCCGGACTCATCGTACTCCTGCTT.
Western blot
The isolation of protein samples was conducted using radio immunoprecipitation assay buffer supplemented with protease inhibitor (catalog number ZP180201, lot number 20220820, Zeye Biotech, Shanghai, China). The following primary antibodies were used (dilution, catalog number, lot number, manufacturer, city, country): Anti-cluster of differentiation (CD) 11b (1:2000, ab128797, lot 230215, Abcam, Cambridge, UK), anti-F4/80 (1:1000, ab100790, lot 221229, Abcam, Cambridge, UK), anti-CD68 (1:1000, ab125212, lot 221103, Abcam, Cambridge, UK), anti- inducible nitric oxide synthase (iNOS) (1:1000, ab178954, lot 221109, Abcam, Cambridge, UK), anti-CD86 (1:1000, ab238468, lot 221011, Abcam, Cambridge, UK) anti-Lama1 (1:1000, PA5-119248, lot 230217, Invitrogen, Carlsbad, CA, USA), anti-Sorcs2 (1:1000, 55063-1-AP, lot 230105, Proteintech, Rosemont, IL, USA), and anti-actin (1:2000, ab8226, lot 220921, Abcam, Cambridge, UK). Secondary antibody: HRP-conjugated goat anti-rabbit immunoglobulin G (IgG) (1:5000, ab6721, lot 221108, Abcam, Cambridge, UK) and HRP-conjugated goat anti-mouse IgG (1:5000, ab205719, lot 221012, Abcam, Cambridge, UK). The development of protein signals was conducted using an enhanced chemiluminescence reagent (catalog number ZP180301, lot number 20220525, Zeye Biotech, Shanghai, China). Densitometric analysis of the resulting bands was performed using ImageJ software (Version 1.53t, NIH, Bethesda, MD, USA).
Flow cytometry
Before antibody staining, cells were pre-treated with Fc receptor blocking solution (anti-CD32, clone 2.4G2, catalog number 550270, lot number 9356780, BD Biosciences, San Jose, CA, USA, 1:500 dilution) for 10 min at 4°C to minimize non-specific binding. Cells were then incubated with a cocktail of fluorophore-conjugated antibodies for 15 min at 4°C. The antibody panel included allophycocyanin-conjugated anti-CD11b (1:600, catalog number 562102, lot 9283645, BD Biosciences, San Jose, CA, USA) and phycoerythrin-conjugated anti-ionized calcium binding adaptor molecule 1 (Iba-1) (1:400, catalog number, 10513S, lot 90298374, Cell Signaling Technologies, Danvers, MA). Following staining, cells were diluted in phosphate-buffered saline, and cell events were recorded using an LSRII flow cytometer (BD Biosciences, San Jose, CA, USA).
Cell growth assay
Cell growth kinetics were examined based on a cell counting kit-8 (CCK-8) kit (catalog number HY-K0301, lot number 221204, MedChemExpress, Shanghai, China). Briefly, cells were initially inoculated at 2,000 cells per well in 96-well plates. At 0, 24, 48, and 72 h post-seeding, 15 μL of CCK-8 reagent was added to each cell sample. After a 2-h reaction duration at 37°C, the absorbance value at 450 nm of each sample was detected using a Synergy H1 microplate reader (Batch number: SH1-2024-BTK-0356; BioTek Instruments, Winooski, VT, USA).
Inflammatory cytokine analysis
The relative levels of tumor necrosis factor-α, interleukin (IL)-1β, and IL-6 were quantified using commercially available enzyme-linked immunosorbent assay (ELISA) kits (catalog numbers: ERC102a.96, ERC007.96, ERC003.96; lot numbers: 20230130, 20230119, 20221202; NeoBioscience, Shanghai, China) following the manufacturer’s protocols. Cytokine levels were derived from standard curves and adjusted for total protein content in each sample, which was measured using a bicinchoninic acid protein assay kit (catalog number 23225, lot number 20230405, Thermo Fisher Scientific, Waltham, MA, USA).
ROS measurement
ROS levels in tissue or cellular specimens were assessed through a specialized ROS assay kit (catalog number E004-1-1, lot number 20230622, Jiancheng Bioengineering Institute, Nanjing, Jiangsu, China). Samples were lysed based on the supplier’s instructions, and 100 μL of each lysate was incubated with 10 μM 2’,7’-Dichlorodihydrofluorescin diacetate (catalog number D6883, lot number MKCN2356, Sigma-Aldrich, St. Louis, MO, USA) for 30 min at 37°C in darkness. The fluorescence signal was measured at excitation/emission: 485/530 nm. Final ROS signals were adjusted to the protein levels within each sample.
Migration assay
To determine cell migratory ability, a scratch wound-healing experiment was conducted. Cells were introduced in a 6-well plate at a density of 15,000 cells/cm2 and cultured until 90% confluence. A linear wound was generated in the middle of cell culture using a 200 μL pipette tip. Wound closure was monitored over 48 h using an inverted light microscope, with images captured at multiple time points.
Statistics
GraphPad Prism version 8.0 (GraphPad Software, San Diego, CA, USA) was adopted for the statistical analyses of the results. Unpaired Student’s t-test was utilized for comparisons between the two groups. Multiple group comparisons were conducted using one-way analysis of variance (ANOVA) followed by Tukey’s post hoc test. Two-way ANOVA was utilized for analyzing results with different time points or factors. Results are reported as mean and standard deviation. The statistical threshold was set at P < 0.05 for all data analyses.
RESULTS
Downregulation of Sorcs2 and Lama1 in the retinal tissues of RIR
We first performed the transcriptome profiles in retinal specimens from the sham group and model group. Transcriptome profiling of retinal tissues from sham and RIR model groups revealed significant downregulation of Sorcs2 and Lama1 genes in the RIR condition [Figure 1a]. These findings were subsequently validated at mRNA and protein levels [Figure 1b and c]. To assess microglial activation, we employed flow cytometry using CD11b and Iba-1 as markers. A notable increase in the CD11b+ Iba-1+ microglial population was observed in retinal tissues following RIR induction [Figure 1d]. This elevation in double-positive cells indicates enhanced microglial activation in response to RIR-induced retinal damage. Collectively, these data demonstrate that RIR injury induces significant alterations in retinal gene expression profiles and promotes microglial activation, potentially contributing to the pathophysiology of retinal damage in this model.
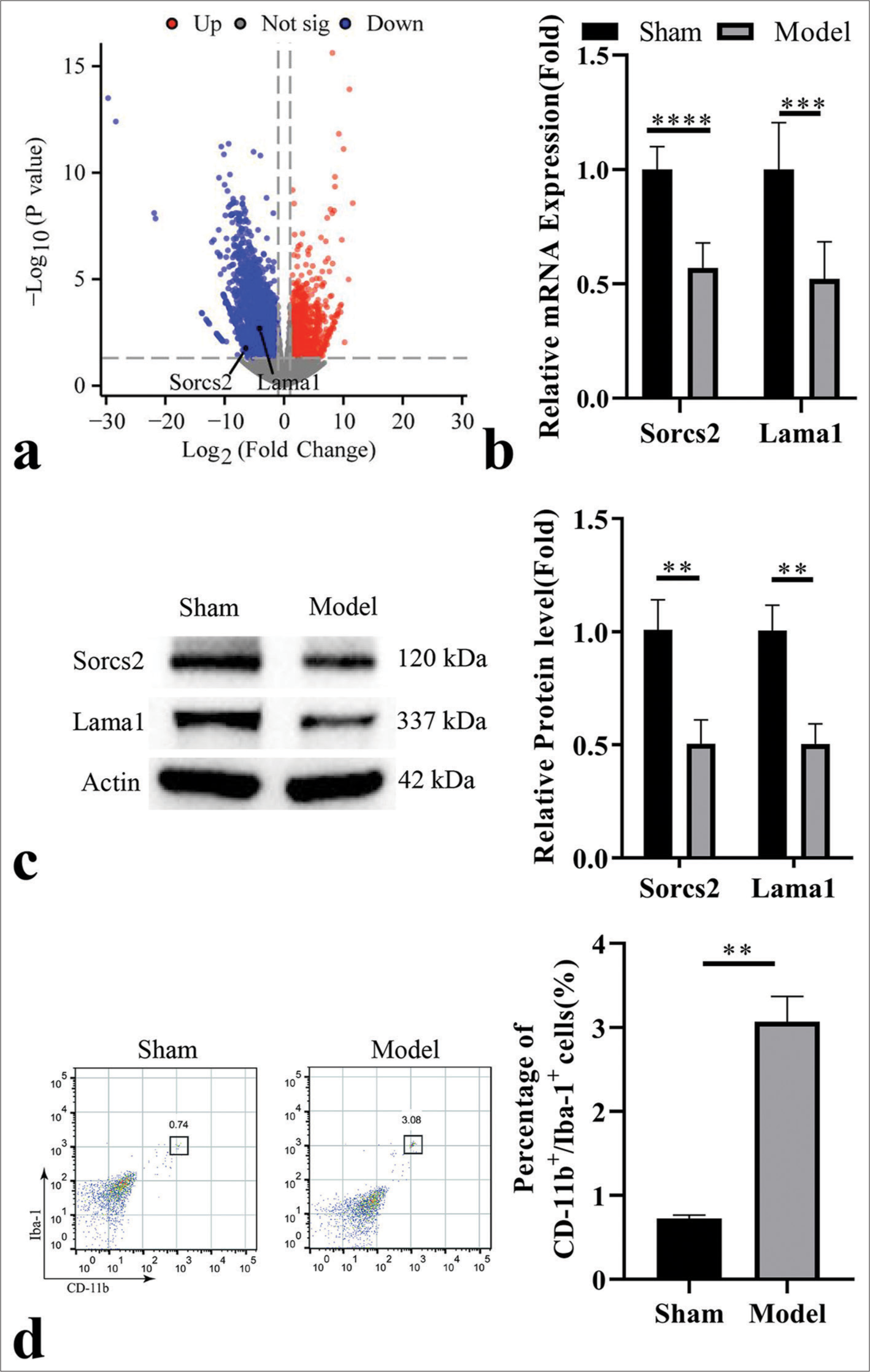
- Downregulation of sortilin-related vacuolar protein sorting 10 domain containing receptor 2 (Sorcs2) and laminin subunit alpha 1 (Lama1) in the retinal tissues of retinal ischemia-reperfusion (RIR). (a) Transcriptome profiles of the retina in the sham and RIR model group. (b) Real-time quantitative polymerase chain reaction and (c) Immunoblotting analysis of Sorcs2 and Lama1 levels in retinal tissues from the sham and RIR model groups. (d) Analysis of cluster of differentiation (CD) 11b + Ionized calcium binding adaptor molecule-1 + microglial cell population in the retinal tissues from the sham and RIR model groups. n = 6 animals per group. ✶P < 0.05; ✶✶P < 0.01; ✶✶✶P < 0.001; ✶✶✶✶P < 0.0001.
OGD/R induces downregulation of Sorcs2/Lama1 and promotes M1 activation of microglia cells
Next, we applied OGD/R in HAPI cells (rat microglia cells) as the cell model of RIR. As expected, OGD/R induction caused the suppression of the growth of HAPI cells [Figure 2a], and the migratory ability of microglia cells was also repressed upon OGD/R induction [Figure 2b]. These was an increased level of ROS production after OGD/R induction [Figure 2c]. Besides, OGD/R induction promoted M1-like polarization of mcroglia, as evidenced by an increase in the expression of M1 markers (CD11b, CD86, and CD68) [Figure 2d]. This was associated with elevated levels of inflammatory factors [Figure 2e]. We also examined the expression of Sorcs2 and Lama1. Similar to their expression pattern in the retinal tissues of RIR, OGD/R induction caused the downregulation of Sorcs2 and Lama1 in microglia cells [Figure 2f and g]. Based on these findings, we further investigated the potential role of Sorcs2 and Lama1 in dictating microglia activation.
![Oxygen-glucose deprivation/reperfusion (OGD/R) induces the downregulation of sortilin-related vacuolar protein sorting 10 domain containing receptor 2 (Sorcs2)/laminin subunit alpha 1 (Lama1) and promotes M1 activation of microglia cells. OGD/R was applied in HAPI cells (rat microglia cells) as a cell model of retinal ischemia-reperfusion. (a) Cell counting kit-8 proliferation assay, (b) Wound-healing migration assay, (c) reactive oxygen species level detection, (d) Western blot analysis of microglia activation markers (inducible nitric oxide synthase, cluster of differentiation [CD]11b, CD86 and CD68), (e) enzyme-linked immunosorbent assay analysis of pro-inflammatory cytokines, (f) real-time quantitative polymerase chain reaction analysis of Sorcs2 and Lama1, and (g) Western blot analysis of Sorcs2 and Lama1 in the control and OGD/R induction groups. n = 3. ✶P < 0.05; ✶✶P < 0.01; ✶✶✶P < 0.001; ✶✶✶✶P < 0.0001. TNF-α: Tumor necrosis factor alpha, IL: Interleukin, ROS: Reactive oxygen species, iNOS: Inducible nitric oxide synthase, OD value: Optical density value.](/content/105/2025/22/1/img/Cytojournal-22-2-g002.png)
- Oxygen-glucose deprivation/reperfusion (OGD/R) induces the downregulation of sortilin-related vacuolar protein sorting 10 domain containing receptor 2 (Sorcs2)/laminin subunit alpha 1 (Lama1) and promotes M1 activation of microglia cells. OGD/R was applied in HAPI cells (rat microglia cells) as a cell model of retinal ischemia-reperfusion. (a) Cell counting kit-8 proliferation assay, (b) Wound-healing migration assay, (c) reactive oxygen species level detection, (d) Western blot analysis of microglia activation markers (inducible nitric oxide synthase, cluster of differentiation [CD]11b, CD86 and CD68), (e) enzyme-linked immunosorbent assay analysis of pro-inflammatory cytokines, (f) real-time quantitative polymerase chain reaction analysis of Sorcs2 and Lama1, and (g) Western blot analysis of Sorcs2 and Lama1 in the control and OGD/R induction groups. n = 3. ✶P < 0.05; ✶✶P < 0.01; ✶✶✶P < 0.001; ✶✶✶✶P < 0.0001. TNF-α: Tumor necrosis factor alpha, IL: Interleukin, ROS: Reactive oxygen species, iNOS: Inducible nitric oxide synthase, OD value: Optical density value.
Sorcs2/Lama1 overexpression suppresses OGD/R-induced M1 activation in microglia cells
HAPI cells were transduced with the lentivirus carrying empty vector, Lama1 or Sorcs2 expression sequence, and cells in different groups were subjected to OGD/R induction. The transduction of lentivirus carrying Lama1 or Sorcs2 could increase the protein expression levels of Lama1 and Sorcs2 after OGD/R induction [Figure 3a]. The overexpression of Lama1 and Sorcs2 partially rescued cell proliferation and cell migratory ability upon OGD/R induction [Figure 3b and c]. However, OGD/R-induced ROS level was not affected after the overexpression of Lama1 and Sorcs2 [Figure 3d]. Further, Western blot and ELISA analysis showed that Sorcs2 or Lama1 overexpression suppressed OGD/R-induced M1 activation and the levels of pro-inflammatory factors in microglia cells [Figure 3e and f]. These data suggest that Sorcs2 and Lama1 are negative regulators of OGD/R-induced M1 activation in microglia cells, but Sorcs2 and Lama1 may function downstream the ROS signaling.
![Sortilin-related vacuolar protein sorting 10 domain containing receptor 2 (Sorcs2)/laminin subunit alpha 1 (Lama1) overexpression suppresses oxygen-glucose deprivation/reperfusion (OGD/R)-induced M1 activation in microglia. HAPI cells were transduced with the lentivirus carrying empty vector, Lama1 or Sorcs2 expression sequence, and cells in different groups were subjected to OGD/R induction. (a) Western blot analysis of Lama1 and Sorcs2; (b) cell counting kit-8 proliferation assay; (c) Wound-healing migration assay; (d) reactive oxygen species level detection; (e) Western blot analysis of microglia activation markers (inducible nitric oxide synthase, cluster of differentiation [CD]11b, CD86 and CD68); (f) Enzyme-linked immunosorbent assay analysis of pro-inflammatory factors. n = 3. ✶P < 0.05; ✶✶P < 0.01; ✶✶✶P < 0.001; ✶✶✶✶P < 0.0001. TNF-α: Tumor necrosis factor alpha, IL: Interleukin, ROS: Reactive oxygen species, iNOS: Inducible nitric oxide synthase, OD value: Optical density value.](/content/105/2025/22/1/img/Cytojournal-22-2-g003.png)
- Sortilin-related vacuolar protein sorting 10 domain containing receptor 2 (Sorcs2)/laminin subunit alpha 1 (Lama1) overexpression suppresses oxygen-glucose deprivation/reperfusion (OGD/R)-induced M1 activation in microglia. HAPI cells were transduced with the lentivirus carrying empty vector, Lama1 or Sorcs2 expression sequence, and cells in different groups were subjected to OGD/R induction. (a) Western blot analysis of Lama1 and Sorcs2; (b) cell counting kit-8 proliferation assay; (c) Wound-healing migration assay; (d) reactive oxygen species level detection; (e) Western blot analysis of microglia activation markers (inducible nitric oxide synthase, cluster of differentiation [CD]11b, CD86 and CD68); (f) Enzyme-linked immunosorbent assay analysis of pro-inflammatory factors. n = 3. ✶P < 0.05; ✶✶P < 0.01; ✶✶✶P < 0.001; ✶✶✶✶P < 0.0001. TNF-α: Tumor necrosis factor alpha, IL: Interleukin, ROS: Reactive oxygen species, iNOS: Inducible nitric oxide synthase, OD value: Optical density value.
OGD/R-induced ROS production mediates the expression of Sorcs2 and Lama1 in M1 activation of microglia cells
We next sought to explore whether OGD/R-induced ROS signaling is responsible for the downregulation of Sorcs2 and Lama1. To this end, we applied N-acetylcysteine (NAC, antioxidant) to neutralize OGD/R-induced ROS in HAPI cells [Figure 4a]. Western blot analysis revealed that the neutralization of ROS under OGD/R condition could restore Sorcs2 and Lama1 expression [Figure 4b]. ROS neutralization also rescued cell proliferation and migration ability after OGD/R induction [Figure 4c and d]. Moreover, the reduction of ROS level attenuated OGD/R-induced M1 activation and pro-inflammatory factor production in microglia cells [Figure 4e and f]. Therefore, these data suggest that OGD/R-induced ROS signaling mediates the downregulation of Sorcs2 and Lama1 and the M1 activation in microglia cells.
![Oxygen-glucose deprivation/reperfusion (OGD/R)-induced reactive oxygen species (ROS) production mediates the expression of sortilin-related vacuolar protein sorting 10 domain containing receptor 2 (Sorcs2) and laminin subunit alpha 1 (Lama1) in M1 activation of microglia cells. N-Acetylcysteine (1 mM, antioxidant) was applied to neutralize OGD/R-induced ROS in HAPI cells for 24 h. (a) ROS level detection; (b) Immunoblotting analysis of Sorcs2 and Lama1 expression; (c) cell counting kit-8 proliferation assay; (d) Wound-healing migration assay; (e) Western blot analysis of microglia activation markers (inducible nitric oxide synthase, cluster of differentiation [CD]11b, CD86 and CD68); (f) Enzyme-linked immunosorbent assay analysis of pro-inflammatory factors. n = 3. ✶P < 0.05; ✶✶P < 0.01; ✶✶✶P < 0.001; ✶✶✶✶P < 0.0001. TNF-α: Tumor necrosis factor alpha, IL: Interleukin, iNOS: Inducible nitric oxide synthase, NAC: N-acetylcysteine, OD value: Optical density value.](/content/105/2025/22/1/img/Cytojournal-22-2-g004.png)
- Oxygen-glucose deprivation/reperfusion (OGD/R)-induced reactive oxygen species (ROS) production mediates the expression of sortilin-related vacuolar protein sorting 10 domain containing receptor 2 (Sorcs2) and laminin subunit alpha 1 (Lama1) in M1 activation of microglia cells. N-Acetylcysteine (1 mM, antioxidant) was applied to neutralize OGD/R-induced ROS in HAPI cells for 24 h. (a) ROS level detection; (b) Immunoblotting analysis of Sorcs2 and Lama1 expression; (c) cell counting kit-8 proliferation assay; (d) Wound-healing migration assay; (e) Western blot analysis of microglia activation markers (inducible nitric oxide synthase, cluster of differentiation [CD]11b, CD86 and CD68); (f) Enzyme-linked immunosorbent assay analysis of pro-inflammatory factors. n = 3. ✶P < 0.05; ✶✶P < 0.01; ✶✶✶P < 0.001; ✶✶✶✶P < 0.0001. TNF-α: Tumor necrosis factor alpha, IL: Interleukin, iNOS: Inducible nitric oxide synthase, NAC: N-acetylcysteine, OD value: Optical density value.
Ectopic expression of Sorcs2 or Lama1 suppresses the pro-inflammatory expansion of microglia cells in the rat model of RIR
To further explore the therapeutic potential of targeting Sorcs2 and Lama1, we administrated the lentivirus carrying empty vector, Lama1 or Sorcs2 expression sequence in the rat before RIR induction. The delivery of Lama1 and Sorcs2 lentivirus restored the expression of Lama1 and Sorcs2 in the retinal tissues, respectively, after RIR induction [Figure 5a]. Forced overexpression of Lama1 or Sorcs2 could suppress the expansion of CD11b + Iba-1+ microglial cell population in RIR-induced retinal tissues [Figure 5b]. Consistently, the elevated levels of pro-inflammatory cytokines after RIR induction were suppressed by the ectopic expression of Sorcs2 or Lama1 [Figure 5c]. However, RIR-induced ROS levels in the retinal tissues were not significantly suppressed upon the ectopic expression of Sorcs2 or Lama1 [Figure 5d].
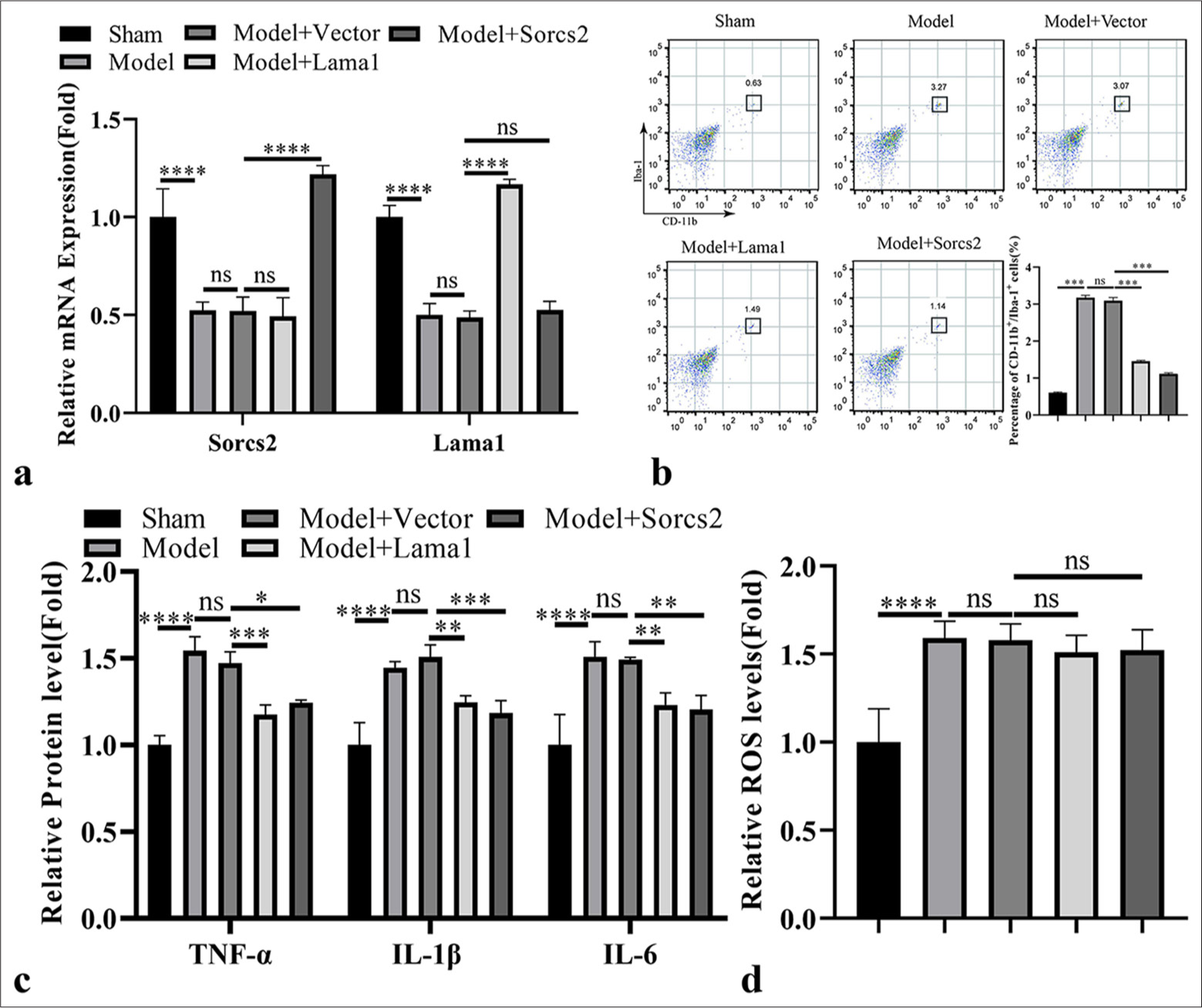
- Ectopic expression of sortilin-related vacuolar protein sorting 10 domain containing receptor 2 (Sorcs2) or laminin subunit alpha 1 (Lama1) suppresses the pro-inflammatory expansion of microglia cells in the rat model of retinal ischemia-reperfusion (RIR). Lentivrius carrying empty vector, Lama1 or Sorcs2 expression sequence was administrated in the rat before RIR induction. (a) Real-time quantitative polymerase chain reaction analysis of Lama1 and Sorcs2 expression in the retinal tissues. (b) Flow cytometry analysis of cluster of differentiation 11b + Ionized calcium binding adaptor molecule-1+ microglial cell population in the retinal tissues. (c) Enzyme-linked immunosorbent assay analysis of pro-inflammatory mediators in the retinal tissues. (d) Reactive oxygen species level detection in the retinal tissues. n = 6 animals per group. ✶P < 0.05; ✶✶P < 0.01; ✶✶✶P < 0.001; ✶✶✶✶P < 0.0001. TNF-α: Tumor necrosis factor alpha, IL: Interleukin, ROS: Reactive oxygen species, iNOS: Inducible nitric oxide synthase, mRNA: Messenger RNA, CD: Cluster of differentiation, ns: Not significant.
AP shows antioxidant effect and suppresses OGD/R-induced M1 activation in microglia cells
AP has been reported with antioxidant and beneficial effects in different pathogenic conditions.[24-26] We next sought to examine whether AP could also prevent OGD/R-triggered ROS generation and microglia cell activation. In OGD/R-treated HAPI cells, AP above 10 μg/mL was able to significantly lower the ROS level [Figure 6a]. The application of AP at 10 and 20 μg/mL partially restored cell proliferation and migration after OGD/R induction [Figure 6b and c]. AP treatment also attenuated OGD/R-induced M1 activation and reduced inflammatory factor levels in microglia cells [Figure 6d and e]. Besides, the attenuation of ROS by AP was accompanied by the elevated expression of Sorcs2 and Lama1 expression [Figure 6f]. These data indicate that the antioxidant effect of AP promotes Sorcs2 and Lama1 expression and suppresses OGD/R-induced M1 activation in microglia cells.
![Astragalus polysaccharide (AP) shows antioxidant effect and suppresses Oxygen-glucose deprivation/reperfusion (OGD/R)-induced M1 activation in microglia cells. In OGD/R-induced HAPI cells, AP was applied at different concentration (10–100 μg/mL) for 24 h. (a) The detection of reactive oxygen species (ROS) level; (b) Cell counting kit-8 (CCK8) proliferation assay; (c) Wound-healing migration assay; (d) Western blot analysis of microglia activation markers (inducible nitric oxide synthase [iNOS], cluster of differentiation [CD]11b, CD86 and CD68); (e) Enzyme-linked immunosorbent assay analysis of pro-inflammatory mediators. (f) Western blot analysis of laminin subunit alpha 1 and sortilin-related vacuolar protein sorting 10 domain containing receptor 2. n = 3. ✶P < 0.05; ✶✶P < 0.01; ✶✶✶P < 0.001; ✶✶✶✶P < 0.0001. TNF-α: Tumor necrosis factor alpha, IL: Interleukin, Lama1: Laminin subunit alpha 1, Sorcs2: Sortilin related vacuolar protein sorting 10 domain containing receptor 2, OD value: Optical density value.](/content/105/2025/22/1/img/Cytojournal-22-2-g006.png)
-
Astragalus polysaccharide (AP) shows antioxidant effect and suppresses Oxygen-glucose deprivation/reperfusion (OGD/R)-induced M1 activation in microglia cells. In OGD/R-induced HAPI cells, AP was applied at different concentration (10–100 μg/mL) for 24 h. (a) The detection of reactive oxygen species (ROS) level; (b) Cell counting kit-8 (CCK8) proliferation assay; (c) Wound-healing migration assay; (d) Western blot analysis of microglia activation markers (inducible nitric oxide synthase [iNOS], cluster of differentiation [CD]11b, CD86 and CD68); (e) Enzyme-linked immunosorbent assay analysis of pro-inflammatory mediators. (f) Western blot analysis of laminin subunit alpha 1 and sortilin-related vacuolar protein sorting 10 domain containing receptor 2. n = 3. ✶P < 0.05; ✶✶P < 0.01; ✶✶✶P < 0.001; ✶✶✶✶P < 0.0001. TNF-α: Tumor necrosis factor alpha, IL: Interleukin, Lama1: Laminin subunit alpha 1, Sorcs2: Sortilin related vacuolar protein sorting 10 domain containing receptor 2, OD value: Optical density value.
AP administration suppresses the pro-inflammatory expansion of microglia cells in the rat model of RIR
Next, we applied AP treatment in the mice of sham and RIR model groups. The administration of AP in the sham group did not affect the percentage of CD11b + Iba-1+ microglial cell population in retinal tissues, while AP treatment significantly reduced the abundance of CD11b + Iba-1+ microglial cells in the RIR group [Figure 7a]. RIR-induced ROS levels in the retinal tissues were also reduced by AP treatment [Figure 7b], and the levels of pro-inflammatory mediators in the retinal tissues after RIR induction were repressed by AP treatment [Figure 7c]. Meanwhile, AP administration also enhanced the expression levels of Sorcs2 and Lama1 [Figure 7d]. Together, our findings imply that the antioxidant activity of AP attenuates the inflammatory activation of microglia cells in the RIR model, possibly through the upregulation of Sorcs2 and Lama1.
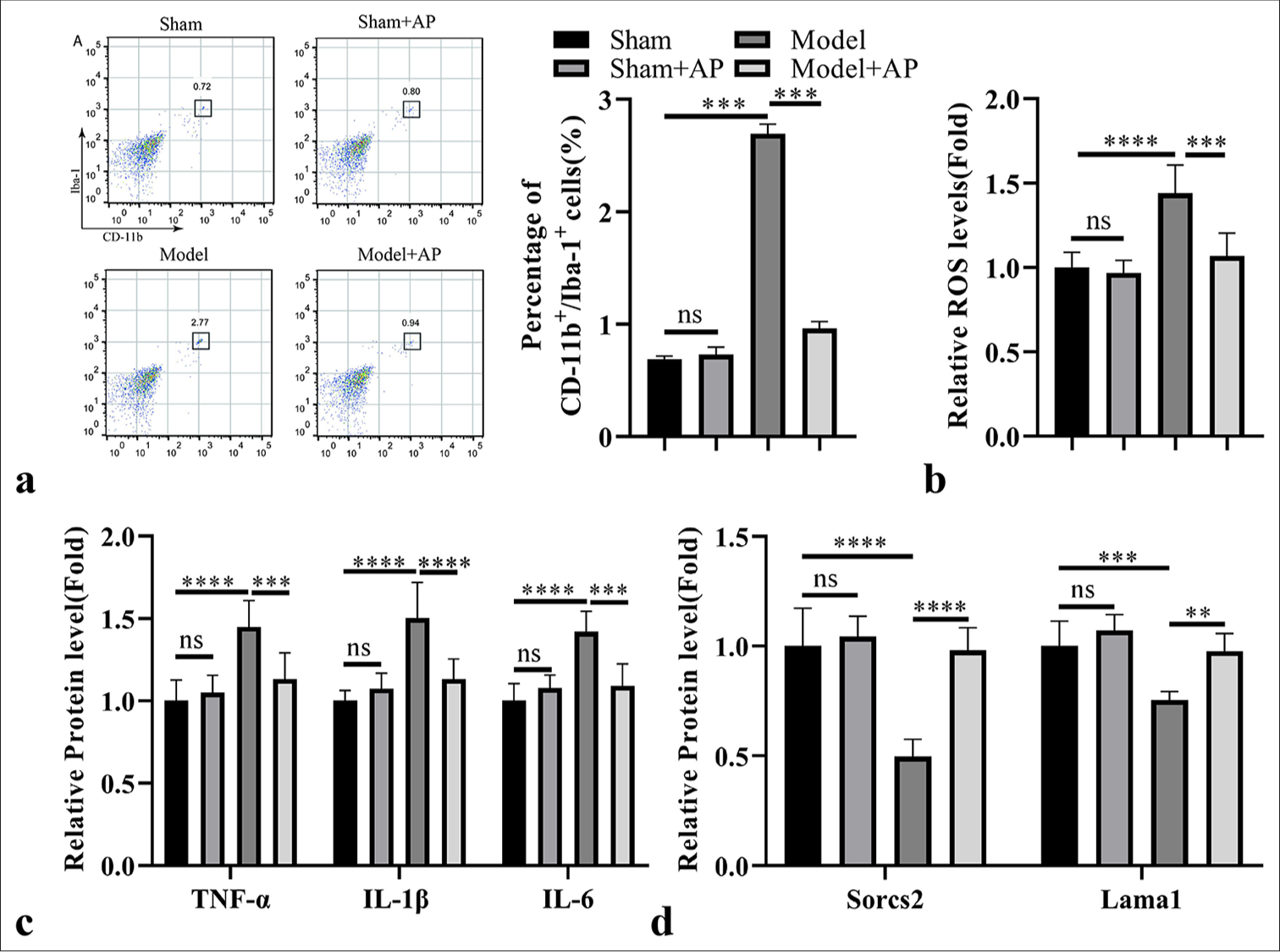
-
Astragalus polysaccharide (AP) administration suppresses the pro-inflammatory expansion of microglia cells in the rat model of retinal ischemia-reperfusion (RIR). AP was administrated in the mice of sham and RIR model groups for 1 week. (a) Analysis of cluster of differentiation (CD) 11b + Ionized calcium binding adaptor molecule-1+ microglial cell population in the retinal tissues. (b) Reactive oxygen species (ROS) level detection in the retinal tissues. (c) Enzyme-linked immunosorbent assay analysis of pro-inflammatory factors in the retinal tissues. (d) RT-qPCR analysis of laminin subunit alpha 1 (Lama1) and sortilin-related vacuolar protein sorting 10 domain containing receptor 2 (Sorcs2) expression in the retinal tissues. n = 6 animals per group. ✶P < 0.05; ✶✶P < 0.01; ✶✶✶P < 0.001; ✶✶✶✶P < 0.0001. TNF-α: Tumor necrosis factor alpha, IL: Interleukin, ns: Not significant.
DISCUSSION
We identified Sorcs2 and Lama1 as two downregulated genes in the retinal tissues of RIR. OGD/R induction caused pro-inflammatory microglial activation, which was accompanied by the downregulation of Sorcs2 and Lama1. Sorcs2 or Lama1 overexpression suppressed OGD/R-induced microglial activation in vitro and also dampened the expansion of microglia in the rat model of RIR. We further showed that AP could neutralize the ROS and promote Sorcs2 and Lama1 expression in OGD/R-treated microglia cells. AP treatment also suppressed microglial pro-inflammatory activation in the rat RIR model.
Microglial activation and polarization into M1-like macrophage state have been widely reported in ischemiareperfusion conditions.[27-29] In agreement with these studies, we showed that there was an expansion of CD11b + Iba+ microglia cells in the retinal tissue after RIR induction. Besides, OGD/R induction also promoted the expression of microglial activation markers (iNOS, CD11b, CD86, and CD68).[30] Our data are also consistent with a recent report that, in a rat model of retinal degeneration, retinal microglia exhibited a notably greater presence of pan-macrophage markers Iba-1+ and F4/80+ cells, along with heightened expression of the activation marker CD68.[31] There is a hypothesis regarding the role of hypoxia in microglial activation under ischemiareperfusion conditions.[32] However, the mechanisms underlying hypoxia-induced microglial activation remain elusive. There is evidence that acute hypoxia promotes the activity of microglia by activating the Nuclear Factor kappa-light-chain-enhancer of activated B cells (NF-κB) signaling.[33] Chronic intermittent hypoxia can induce the generation of damage-associated molecule patterns, which activate toll-like receptor signaling in microglia.[34] However, the activation of hypoxia-inducible factor under hypoxia has also been reported to induce microglial quiescence and cell death possibly due to the compromised mitochondrial metabolism.[35,36]
In our study, we reported Sorcs2 and Lama1 as two genes being downregulated in microglial activation under RIR conditions. Sorcs2 or Lama1 overexpression was able to dampen the inflammatory activation of microglial activation in the cell and rat model of RIR. Sorcs2 has been characterized as the family member of vacuolar protein sorting 10 domain-containing receptors that bind to neuronal growth factors.[37] Its family member sorting protein-related receptor with A-type repeats has recently been implicated in the regulation of monocyte migration through IL-6-mediated signaling.[38] Lama 1 encodes the alpha 1 subunit of laminin, a key glycoprotein protein in the extracellular matrix.[39] A previous study reported that silencing Lama1 could attenuate the alternative and fibrotic macrophage activation in pulmonary fibrosis, indicating a functional engagement of Lama1 in macrophage polarization.[40] Although the mechanisms by which Sorcs2 and Lama1 control the polarization of microglia (macrophages) are unclear, our data highlighted these two genes as negative regulators of microglial activation.
We further showed that RIR-induced ROS is an upstream factor responsible for the downregulation of Sorcs2 and Lama1 since neutralizing oxidative stress could restore the expression of Sorcs2 and Lama1 in microglia after RIR induction. There is evidence that ROS can modulate their expression.[41,42] For instance, loss of Sorcs2 expression can be induced by ROS-induced DNA double-strand breaks.[42] Since ROS orchestrates a variety of cellular signaling pathways,[43] future work is warranted to clarify further the underlying signaling events responsible for ROS-dependent Sorcs2 and Lama1 downregulation in microglia.
AP has been widely reported with antioxidant effects and has been demonstrated with therapeutic effects in pathogenic conditions associated with oxidative stress.[24-26] Besides, AP also shows anti-inflammatory effects in animal and cell models of inflammatory conditions.[44-47] We provided novel evidence supporting the antioxidant effect of AP in preventing microglial activation under RIR conditions. The ROS neutralization by AP treatment upregulated Sorcs2 and Lama1 levels and suppressed microglial pro-inflammatory activation. Since microglia are tissue-resident macrophages and the inflammatory polarization of macrophages is implicated in the inflammatory progression of multiple pathogenic conditions, it is anticipated that AP could be applied as a wide-spectrum antioxidant and anti-inflammatory agent to improve inflammatory conditions.[48]
While our study offers valuable insights into the role of Sorcs2 and Lama1 in RIR injury and the therapeutic potential of AP, several limitations should be acknowledged. First, although the OGD/R model is well established for studying ischemic conditions, its direct relevance to human RIR conditions needs further exploration. Further studies are needed to validate these findings in more advanced models or human tissue samples. Second, the mechanisms through which AP exerts its effects on microglial activation remain elusive. Further research is needed to determine whether AP’s effects are direct or indirect and to identify other potential pathways involved. This could be addressed by conducting comprehensive omics studies, such as transcriptomics and proteomics, to identify the signaling pathways regulated by AP. Once potential pathways are identified, specific inhibitors or activators could be applied to validate their involvement in AP’s effects on microglial activation. In addition, the study reports that AP promotes the expression of Sorcs2 and Lama1, but the precise mechanisms of this upregulation are not fully elucidated. Future work should focus on identifying the transcription factors or signaling pathways involved in AP-mediated upregulation of these genes, possibly through chromatin immunoprecipitation assays or promoter analysis studies. Such investigations could provide deeper insights into the molecular mechanisms underlying AP’s therapeutic potential and may reveal new targets for intervention in RIR injury.
SUMMARY
To sum up, Sorcs2 and Lama1 are two potential negative regulators of microglial activation in RIR. The antioxidant effect of AP could neutralize RIR-induced ROS, promote Sorcs2 and Lama1 expression, and suppress the pro-inflammatory activation of microglia cells. AP may be employed as an antioxidant to attenuate microglial activation and ameliorate the inflammatory damages in RIR.
AVAILABILITY OF DATA AND MATERIALS
The data and materials generated in this study are available upon request to the corresponding author. The cell line and other materials used in the study are available upon reasonable request.
ABBREVIATIONS
APC: Allophycocyanin
AP: Astragalus polysaccharide
BCA: Bicinchoninic acid
CCK-8: Cell counting kit-8
CD11b: Cluster of Differentiation 11b
CD32: Cluster of Differentiation 32
CD68: Cluster of Differentiation 68
CD86: Cluster of Differentiation 86
DAMPs: Damage-associated molecule patterns
DMEM: Dulbecco’s modified eagle medium
ECL: Enhanced chemiluminescence
ELISA: Enzyme-linked immunosorbent assay
HIF: Hypoxia inducible factor
Iba-1: Ionized calcium binding adaptor molecule 1
IgG: Immunoglobulin G
IL-1β: Interleukin-1β
IL-6: Interleukin-6
iNOS: inducible Nitric Oxide Synthase
Lama1: Laminin subunit alpha 1
NAC: N-Acetylcysteine
NF-κB: Nuclear Factor kappa-light-chain-enhancer of activated B cells
OGD/R: Oxygen-glucose deprivation/reperfusion
PBS: Phosphate-buffered saline
PE: Phycoerythrin
RGCs: Retinal ganglion cells
RIR: Retinal ischemia-reperfusion
RIPA: Radio immunoprecipitation assay
ROS: Reactive oxygen species
RT-qPCR: Real-time quantitative polymerase chain reaction
SD: Standard deviation
Sorcs2: Sortilin-related Vacuolar Protein Sorting 10 Domain Containing Receptor 2
TLR: Toll-like receptor
TNF-α: Tumor necrosis factor-α
VPS10: Vacuolar protein sorting 10
AUTHOR CONTRIBUTIONS
JJL and ZKZ: Participated in research design; HL, CLW, and ZKZ: Conducted experiments; CLW, CC, and HL: Performed data analysis; CC and JJL: Wrote or contributed to the writing of the manuscript. All authors approved the final version of the manuscript for publication. All authors participated fully in the work, took public responsibility for appropriate portions of the content, and agreed to be accountable for all aspects of the work in ensuring that questions related to the accuracy or completeness of any part of the work were appropriately investigated and resolved.
ACKNOWLEDGMENT
Not applicable.
ETHICS APPROVAL AND CONSENT TO PARTICIPATE
Animal protocols were in compliance with the guidelines of animal care and welfare. All procedures followed protocols approved by Yunnan University’s Laboratory Animal Welfare Ethics Committee (approval number: YNU20240893), dated 04/10/2024. Informed consent was not required as no patients were involved in this study.
CONFLICT OF INTEREST
The authors declare no conflict of interest.
EDITORIAL/PEER REVIEW
To ensure the integrity and highest quality of CytoJournal publications, the review process of this manuscript was conducted under a double-blind model (authors are blinded for reviewers and vice versa) through an automatic online system.
FUNDING
This work was supported by the Medical Discipline Leader Training Program of Yunnan Province Health Commission (Grant No.: D-2019021).
References
- Neuroprotective and anti-inflammatory activities of hybrid small-molecule SA-10 in ischemia/reperfusion-induced retinal neuronal injury models. Cells. 2024;13:396.
- [CrossRef] [PubMed] [Google Scholar]
- Metformin protects against retinal ischemia/reperfusion injury through AMPK-mediated mitochondrial fusion. Free Radic Biol Med. 2023;205:47-61.
- [CrossRef] [PubMed] [Google Scholar]
- Impairments of retinal hemodynamics and oxygen metrics in ocular hypertension-induced ischemia-reperfusion. Exp Eye Res. 2022;225:109278.
- [CrossRef] [PubMed] [Google Scholar]
- Reactive oxygen species-responsive mitochondria-targeted liposomal quercetin attenuates retinal ischemia-reperfusion injury via regulating SIRT1/FOXO3A and p38 MAPK signaling pathways. Bioeng Transl Med. 2022;8:e10460.
- [CrossRef] [PubMed] [Google Scholar]
- Withaferin a attenuates retinal ischemia-reperfusion injury via Akt-dependent inhibition of oxidative stress. Cells. 2022;11:3113.
- [CrossRef] [PubMed] [Google Scholar]
- Inflammatory resolution and vascular barrier restoration after retinal ischemia reperfusion injury. J Neuroinflammation. 2021;18:186.
- [CrossRef] [PubMed] [Google Scholar]
- N,N-Dimethyl-3β-hydroxycholenamide attenuates neuronal death and retinal inflammation in retinal ischemia/reperfusion injury by inhibiting Ninjurin 1. J Neuroinflammation. 2023;20:91.
- [CrossRef] [PubMed] [Google Scholar]
- An overview of ischemia-reperfusion injury: Review on oxidative stress and inflammatory response. Eurasian J Med. 2022;54(Suppl 1):62-5.
- [Google Scholar]
- N-acetylserotonin alleviates retinal ischemia-reperfusion injury via HMGB1/RAGE/NF-κB pathway in rats. Int J Ophthalmol. 2024;17:228-38.
- [CrossRef] [PubMed] [Google Scholar]
- Inhibition of retinal ischemia-reperfusion injury in rats by inhalation of low-concentration hydrogen gas. Graefes Arch Clin Exp Ophthalmol. 2024;262:823-33.
- [CrossRef] [Google Scholar]
- A mouse model of retinal ischemia-reperfusion injury through elevation of intraocular pressure. J Vis Exp. 2016;113:54065.
- [CrossRef] [Google Scholar]
- Inactivation of endothelial ADAM17 reduces retinal ischemia-reperfusion induced neuronal and vascular damage. Int J Mol Sci. 2020;21:5379.
- [CrossRef] [PubMed] [Google Scholar]
- Retinal degeneration induced in a mouse model of ischemiareperfusion injury and its management by pemafibrate treatment. FASEB J. 2022;36:e22497.
- [CrossRef] [PubMed] [Google Scholar]
- The role of microglia in inherited retinal diseases. Adv Exp Med Biol. 2023;1415:197-205.
- [CrossRef] [PubMed] [Google Scholar]
- Retinal microglia-A key player in healthy and diseased retina. Prog Neurobiol. 2019;173:18-40.
- [CrossRef] [PubMed] [Google Scholar]
- Microglia preserve visual function loss in the aging retina by supporting retinal pigment epithelial health. Immun Ageing. 2023;20:53.
- [CrossRef] [PubMed] [Google Scholar]
- Microglia activation and inflammation during the death of mammalian photoreceptors. Annu Rev Vis Sci. 2020;6:149-69.
- [CrossRef] [PubMed] [Google Scholar]
- Microglia versus monocytes: Distinct roles in degenerative diseases of the retina. Trends Neurosci. 2020;43:433-49.
- [CrossRef] [PubMed] [Google Scholar]
- Macrophages coordinate immune response to laser-induced injury via extracellular traps. J Neuroinflammation. 2024;21:68.
- [CrossRef] [PubMed] [Google Scholar]
- Retinal microglia: Functions and diseases. Immunology. 2022;166:268-86.
- [CrossRef] [PubMed] [Google Scholar]
- Retinal ischemia triggers early microglia activation in the optic nerve followed by neurofilament degeneration. Exp Eye Res. 2020;198:108133.
- [CrossRef] [PubMed] [Google Scholar]
- The roles of microglia in neural remodeling during retinal degeneration. Histol Histopathol. 2022;37:1-10.
- [Google Scholar]
- Early-life peripheral infections reprogram retinal microglia and aggravate neovascular age-related macular degeneration in later life. J Clin Invest. 2023;133:e159757.
- [CrossRef] [PubMed] [Google Scholar]
- Regulation and mechanism of Astragalus polysaccharide on ameliorating aging in Drosophila melanogaster. Int J Biol Macromol. 2023;234:123632.
- [CrossRef] [PubMed] [Google Scholar]
- Astragalus polysaccharides protect against dextran sulfate sodium-induced colitis by inhibiting NF-κB activation. Int J Biol Macromol. 2017;98:723-9.
- [CrossRef] [PubMed] [Google Scholar]
- Astragalus polysaccharide: A review of its immunomodulatory effect. Arch Pharm Res. 2022;45:367-89.
- [CrossRef] [PubMed] [Google Scholar]
- Microglia activation in retinal ischemia triggers cytokine and toll-like receptor response. J Mol Neurosci. 2021;71:527-44.
- [CrossRef] [PubMed] [Google Scholar]
- Thymoquinone regulates microglial M1/M2 polarization after cerebral ischemia-reperfusion injury via the TLR4 signaling pathway. Neurotoxicology. 2024;101:54-67.
- [CrossRef] [PubMed] [Google Scholar]
- Analgecine regulates microglia polarization in ischemic stroke by inhibiting NF-κB through the TLR4 MyD88 pathway. Int Immunopharmacol. 2021;99:107930.
- [CrossRef] [PubMed] [Google Scholar]
- Regulation of microglial activation by Wnt/β-catenin signaling after global cerebral ischemia in mice. Mol Neurobiol. 2024;61:308-25.
- [CrossRef] [PubMed] [Google Scholar]
- Single cell RNA sequencing confirms retinal microglia activation associated with early onset retinal degeneration. Sci Rep. 2022;12:15273.
- [CrossRef] [PubMed] [Google Scholar]
- Roles of activated microglia in hypoxia induced neuroinflammation in the developing brain and the retina. J Neuroimmune Pharmacol. 2013;8:66-78.
- [CrossRef] [PubMed] [Google Scholar]
- Hypoxia-induced activation of HIF-1alpha/IL-1beta axis in microglia promotes glioma progression via NF-κB-mediated upregulation of heparanase expression. Biol Direct. 2024;19:45.
- [CrossRef] [PubMed] [Google Scholar]
- Microglia at the crossroads of pathogen-induced neuroinflammation. ASN Neuro. 2022;14:17590914221104566.
- [CrossRef] [PubMed] [Google Scholar]
- Role of hypoxiainducible factor1α in autophagic cell death in microglial cells induced by hypoxia. Mol Med Rep. 2017;15:2097-105.
- [CrossRef] [PubMed] [Google Scholar]
- Hypoxia compromises the mitochondrial metabolism of Alzheimer's disease microglia via HIF1. Nat Aging. 2021;1:385-99.
- [CrossRef] [PubMed] [Google Scholar]
- Structural insights into SorCS2-nerve growth factor complex formation. Nat Commun. 2018;9:2979.
- [CrossRef] [PubMed] [Google Scholar]
- Sortilin expression levels and peripheral immunity: A potential biomarker for segregation between Parkinson's disease patients and healthy controls. Int J Mol Sci. 2024;25:1791.
- [CrossRef] [PubMed] [Google Scholar]
- Increased expression of laminin subunit alpha 1 chain by dCas9-VP160. Mol Ther Nucleic Acids. 2017;6:68-79.
- [CrossRef] [PubMed] [Google Scholar]
- Laminin α1 is a genetic modifier of TGF-β1-stimulated pulmonary fibrosis. JCI Insight. 2018;3:e99574.
- [CrossRef] [PubMed] [Google Scholar]
- Sortilin drives hypertension by modulating sphingolipid/ceramide homeostasis and by triggering oxidative stress. J Clin Invest. 2022;132:e156624.
- [CrossRef] [PubMed] [Google Scholar]
- Loss of SORCS2 is associated with neuronal DNA double-strand breaks. Cell Mol Neurobiol. 2023;43:237-49.
- [CrossRef] [PubMed] [Google Scholar]
- Reactive oxygen species and cell signaling. Review. Biochim Biophys Acta Mol Cell Res. 2024;1871:119573.
- [CrossRef] [PubMed] [Google Scholar]
- Identifying the anti-inflammatory effects of astragalus polysaccharides in anti-N-Methyl-D-aspartate receptor encephalitis: Network pharmacology and experimental validation. Comb Chem High Throughput Screen. 2024;27:1022-32.
- [CrossRef] [PubMed] [Google Scholar]
- Repurposing astragalus polysaccharide PG2 for inhibiting ACE2 and SARS-CoV-2 spike syncytial formation and anti-inflammatory effects. Viruses. 2023;15:641.
- [CrossRef] [PubMed] [Google Scholar]
- Astragalus polysaccharide protects experimental colitis through an aryl hydrocarbon receptor-dependent autophagy mechanism. Br J Pharmacol. 2024;181:681-97.
- [CrossRef] [PubMed] [Google Scholar]
- Astragalus polysaccharides alleviates LPS-induced inflammation via the NF-κB/MAPK signaling pathway. J Cell Physiol. 2020;235:5525-40.
- [CrossRef] [PubMed] [Google Scholar]
- M1/M2 macrophages and their overlaps-myth or reality? Clin Sci (Lond). 2023;137:1067-93.
- [CrossRef] [PubMed] [Google Scholar]