Translate this page into:
Cell-blocks and other ancillary studies (including molecular genetic tests and proteomics)
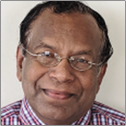
-
Received: ,
Accepted: ,
How to cite this article: Shidham VB. Cell-blocks and other ancillary studies (including molecular pathology and proteomics). CytoJournal 2021;18:4.
HTML of this article is available FREE at: https://dx.doi.org/10.25259/Cytojournal_3_2021.
Abstract
Many types of elective ancillary tests may be required to support the cytopathologic interpretations. Most of these tests can be performed on cell-blocks of different cytology specimens. The cell-block sections can be used for almost any special stains including various histochemistry stains and for special stains for different microorganisms including fungi, Pneumocystis jirovecii (carinii), and various organisms including acid-fast organisms similar to the surgical biopsy specimens. Similarly, in addition to immunochemistry, different molecular tests can be performed on cell-blocks. Molecular tests broadly can be divided into two main types Molecular genetic tests and Proteomics.
Keywords
CellBlockistry
Proteomics
Cell blocks
Cellblocks
Cell-blocks
Cell-blocking
Cell-blocked
NextGen CelBloking
FFPE
Molecular
PCR
FISH
CISH
Genomics
Epigenomics
Transcriptomics
miRNomics

A variety of elective ancillary studies required to support and refine the cytopathologic interpretation of various cytology specimens can be performed on cell-blocks with many benefits[1,2] including multiple advantages due to the application comparable to the Subtractive Coordinate Immunoreactivity Pattern (SCIP) approach.[3] To list a few, the types of ancillary studies which can be performed on cell-blocks include special stains for organisms and other different histochemistry tests, immunohistochemistry (IHC) with SCIP approach, and many types of molecular studies [Figures 1-3].[3] The application of IHC on cell-blocks is discussed in detail in other reviews.[3a,3b]

- Orange-yellow birefringence (red arrowheads in ‘a’ and ‘c’) under polarized light. The color changes to apple green (red arrowheads in ‘b’ and ‘d’) when the axis of the polarizer (blue arrows) is changed by 90 degrees. [10 μ thick sections stained with Congo red: (a and b) Cell-block of fat pad aspirate; (c and d) Amyloid positive control].

- Steps in PCR and reverse transcriptase (RT)-PCR: (a) Denaturing: Two strands of the helix of the target genetic material are unwound and separated by heating. (b) Annealing (hybridization): oligonucleotide primers bind to their complementary bases on the single-stranded DNA. (c) Polymerization: The polymerase enzyme reads the template strand and matches it with the appropriate nucleotides, resulting in two new identical helixes. This results in millions of identical copies of the original DNA or RNA sequence after 30–40 cycles.

- Various molecular tests [Molecular genetic tests (A through D) and Proteomics (E)] on FFPE of cell-blocks.
Special stains
Similar to the surgical biopsy specimens, the cell-block sections can be used for almost any special stains for microorganisms including fungi, Pneumocystis jirovecii (carinii), and various organisms including different acid-fast organisms.[4]
Although the role of histochemistry, in general, is declining due to refinements in IHC technology, there are a significant number of clinical scenarios when histochemistry is still indicated. Most of the histochemistry special stains which can be performed on Formalin-fixed paraffin-embedded (FFPE) of surgical pathology specimens discussed in some dedicated reviews,[5] can also be performed on FFPE of cell-blocks. The list of histochemical stains that may usually be indicated on cytopathology specimens includes iron stain, melanin bleach stain, and PAS stain. Congo red stain with polarizing microscopy [Figure 1][6] and similar amyloid stains may also be performed on sections of FFPE of cell-block for amyloid.
Molecular tests
As described by National Cancer Institute: “In medicine, a laboratory test that checks for certain genes, proteins, or other molecules in a sample of tissue, blood, or other body fluid. Molecular tests also check for certain changes in a gene or chromosome that may cause or affect the chance of developing a specific disease or disorder, such as cancer. A molecular test may be done with other procedures, such as biopsies, to help diagnose some types of cancer. It may also be used to help plan treatment, find out how well the treatment is working, or make a prognosis” [Table 1].[7]
Test and method | Performed on | Strength/Limitation | Benefits |
---|---|---|---|
bioT3 Metastatic Cancer Solution[78] includes CancerTYPE ID®(92-gene real-time RT-PCR assay) and CancerTreatment NGS+[74,75](bio Theranostics, Inc., San Diego, CA, USA) | Formalin-fixed paraffin-embedded | Need at least 300 tumor cells (exclude decalcified bone samples) | • Identifies primary site in 85% of cases with failure in 13% (usually due to insufficient tumor component) • Allows exclusion of some primary sites • Commonly recognized primary sites include biliary, urothelial, colorectal, and pulmonary • Depending on the type of primary site, the median survival is improved[79,80] |
microRNA expression pattern[72,73] | Formalin-fixed paraffin-embedded | miRNA are more resistant to extreme temperatures and pH | • microRNA (miRNA) are non-coding RNA with approximately 22 nucleotides • miRNA regulates many mRNA transcripts • Based on various studies, different primary sites have a distinct miRNA expression pattern |
Epigenetic profiling EPICUP®[76] The microarray DNA methylation signature (Bellvitge Biomedical Research Institute, Barcelona, Spain) |
Formalin-fixed paraffin-embedded | DNA is stableirrespective of tissue fixation[81] | • A system in which a test DNA methylation fingerprint is compared with a database of different tumor primaries • Assay showed good specificity and sensitivity • Primary site identified in 87% of cases with unknown primary • Type-specific therapy improved the overall survival as compared to empiric therapy • Common primary sites include pulmonary, bile ducts, upper aerodigestive tract, breast, colon, liver, and pancreas (in that order) |
FoundationOne CDx™(F1CDx™)[77] Next-generation sequencing analysis of 324 genes. (Foundation Medicine, Cambridge, MA, USA) |
Formalin-fixed paraffin-embedded | A mutation below the detection limits may lead to false-negative results Detection of copy number alterations such as for ERBB2 may decrease in specimens with tumor components less than 25% |
• Detects substitutions, insertion and deletion alterations (indels), and copy number alterations • Identifies genetic alterations (about 4 per tumor) which may have therapeutic benefits in about 85% of cases with clinical benefits[82] • Although confirming the primary site is important because a particular mutation may differ from one primary site to another, this test does not type for the primary site |
For the following review, it is further divided into two main types of testing [Figure 3]:
Molecular genetic tests
Proteomics
Molecular genetic tests [Figure 3A through D]
Application of cytology preparations may show suboptimal DNA because of many pre-analytical variables; however, cell-blocks showed better DNA yield as compared to other cytology preparations.[8] Because of this, good quality cell-blocks of any cytology specimen is a preferred option for different molecular studies such as next-generation sequencing (NGS).[8] Because cell-block sections also allow concurrent morphological evaluation, they are suitable for morphology-based molecular studies such as Fluorescent in situ hybridization (FISH) and Chromogenic in situ hybridization (CISH) for various indications.[9-12]
The limiting factors in molecular testing on cell-blocks include quantitative optimacy of the cell-block with an absolute and relative abundance of diagnostic tumor cells or lesional cells. With ongoing advances in molecular techniques, many molecular studies can be performed on a relatively few number of diagnostic cells. However, some tests are limited by the noise related to the proportion of contaminant non-tumor cells.
Cytopathology specimens predominantly affected by the limitation related to noise include effusion specimens with a significant number of reactive mesothelial cells and inflammatory cells diluting the diagnostic tumor cells in the effusion specimen. Other specimens in this category include Endoscopic ultrasound guided fine needle aspiration (EUS-FNA) specimens from respiratory and gastrointestinal sites with contaminant cells from respective mucosae. The proportion of diagnostic tumor cells in such specimens in comparison to the non-tumor nucleated cells would dictate if a particular molecular test can be performed without micro-dissection. Because of this, it is important to document in the cytopathology report (in case molecular test may be indicated at a later stage), to include a note about the relative proportion of tumor cells as compared to other non-tumor nucleated cells in the cell-block. However, in cases with relatively few diagnostic tumor cells with a proportion lower than that needed for a particular molecular test, laser capture microdissection may be applied to dissect the diagnostic cells in the number required for that particular protocol.[13]
Cytopathologists and pathologists play a significantly critical role in the optimum management by providing proper details on cell-block components for elective molecular pathology testing as indicated.[14] The leading factor in up to 25% of the cases responsible for technical failure in molecular testing is an insufficient number of tumor cells.
The most significant benefit of cell-blocks as compared to the core biopsies is that the cell-blocks predominantly contain the diagnostic tumor cells without stroma present in surgical biopsies [Figures 4 and 5]. Due to this, properly made quantitatively and qualitatively optimum cell-blocks should be the preferred approach for performing most of the molecular tests. As mentioned previously, even the hypocellular cell-blocks may be appropriate in concert with laser capture microdissection.
The role of molecular testing on cell-blocks to choose appropriate therapeutic options with the improved patient outcome has been well recognized. Molecular testing in general is an expensive option and its beneficial role is still evolving. As a result, the application of molecular testing is usually performed in selected cases.
As the role of molecular testing is increasing, diagnostic tumor cells may be required in many more cases in the coming years. This highlights the significance of communicating the possibility of performing molecular tests on the cell-block in the requisition clearly, so that the pathologist evaluating the tissue will try to conserve the utilization of cell-block while performing routine ancillary tests such as IHC. With reference to this critical consideration, the cell-block material should be used frugally for ancillary tests as a routine caution. Similarly, although the molecular testing may not be required as an immediate indication, it may have to be performed in the distant future[15] with ongoing advances in personalized cancer therapy.[16] Cell-blocks would be and may be the only archived resource for molecular tests at a later date.
General considerations
As discussed above, the molecular test can be performed on a variety of cytology specimens.[17,18] However, many variables including institution protocol, clinical setting, handling/ processing from collection to testing, and methodologies applied may lead to suboptimal outcomes. NGS platforms are usually the preferred approach for testing. Although amplification-based NGS assays can be executed with relatively less input sample to test a limited genomic territory, the requirement for a higher quantity of DNA may not be possible with a small number of diagnostic components in cell-blocks and tiny tissue biopsies without following well-planned testing and handling protocol.
The approaches include proper utilization of supernatant material in cytologic specimens for some molecular tests to the establishment of proper harvesting of diagnostic material with properly prepared cell-blocks. Besides, innovative approaches to procure the maximum amount of nucleic acids such as the application of mineral oil to deparaffinize the cell-blocks with the introduction of optimum molecular laboratory process and enhanced application of bioinformatics have increased the proportion of cases with higher success rates.
It is important to recognize the significance and broad ramifications related to the importance of interdisciplinary participation as a cohesive component associated with clinical and provider staff, surgical pathology and cytopathology staff, molecular pathology lab, and bioinformatics personnel.
Fine-needle aspirates achieve sampling higher proportion of diagnostic cells with a higher tumor to stroma ratio as compared to the core needle biopsies and surgical pathology specimens [Figures 4 and 5].[19] Because of this, a quantitatively small amount of Fine-needle aspiration (FNA) sample yields better quality of nucleic acid with less noise than in the core needle biopsies. This feature extends significant advantage during genomic analysis.

- Core biopsy versus Fine Needle Aspiration Biopsy (FNAB): (a) The needle biopsy cores out everything in the path of the needle including the stroma which may be the predominant component of many tumors such as most pancreatic adenocarcinomas. (b) The core is processed to get FFPE, the sections of which may not even have the diagnostic tumor cells in some levels, especially in very narrow tiny core biopsies. (c) In contrast, the FNAB procedure predominantly aspirates relatively discohesive, diagnostic tumor cells without aspirating the stringy, tighter stroma. (d) Besides, the FNAB procedure would sample different areas of lesion than only a few tracts by the core biopsy. The outcome is the desired sampling of predominantly diagnostic tumor cells [see also Figure 5] representing relatively wider areas of the lesion.

- Sampling pattern with FNAB as obvious in cell-blocks with the predominance of diagnostic tumor cells without stroma (HE stain, Cell-block section of EUS-FNAB needle rise of pancreatic adenocarcinoma, cell-block made with plasma-thrombin method).
A commonly studied example while applying various parameters of molecular testing is lung adenocarcinoma. In general, cytology specimens are the default sample available for molecular testing in most of these cases. The field of targetable biomarkers is advancing continuously with the ongoing identification of numerous genetic changes in various tumors. Such changes include point mutations, structural chromosomal rearrangements, insertions, deletions, and changes in copy number. NGS assays are increasingly adopted because they require less DNA in limited diagnostic material. Many animated presentations on web describe the NGS methodology for the beginners.[19-22] However, this method has limitations if all the parameters mentioned above such as point mutations, structural chromosomal rearrangements, insertions, deletions, and change in copy number have to be evaluated concurrently. This is less of an issue these days because of significant advancements in library preparation and NGS data analysis that is better able to detect various types of genetic abnormalities including structural rearrangements.
During the initial phases of molecular testing of lung carcinoma for EGFR analysis, high success rates were reported on routine cytology specimens without significant changes in routine cytology and molecular laboratory protocols.[23] However, most of the cytology samples and small biopsies may not be suitable for more comprehensive genomic profiling with hybrid capture-based NGS assays which usually require a relatively larger quantity of nucleic acid input.[12] Various approaches have been evaluated and reported for handling cytology samples for maximizing the DNA yield for the comprehensive hybrid capture-based assays.[18] The efforts to improve the results with the best outcome from FFPE of cell-blocks can be applied at various stages in the molecular testing workflow during the following steps:
Improve cytology processing protocols with the molecular test in mind
Processing of cell-blocks for the highest yield of diagnostic tumor cells
Organize molecular laboratory for cytology specimens in the algorithm
Update bioinformatics services for any changes such as modified extraction process.
i. Improve cytology processing protocols with the molecular test in mind
FFPE of cell-blocks provides an excellent source of diagnostic material without significant stromal contamination for many ancillary studies including a molecular test on cytology specimens. Guidelines from the College of American Pathologists, International Association for the Study of Lung Cancer, and Association for Molecular Pathology recommend FFPE of cell-blocks for molecular studies for lung carcinoma as a preferred specimen type.[24]
This recognizes the fact that just processing for quantitatively and qualitatively optimum cell-block may not be enough while processing the cytopathology specimen. Based on the principle of maximum utilization of minimum resources, most of the components of the cytology specimen should be utilized. In addition, it has been reported that the supernatants of cytology specimens have a considerable amount of nucleic acid after separating the sediments by centrifugation.[25] Depending on the laboratory protocol and clinical scenario, the supernatant could also be properly triaged.
The DNA extracted from the supernatant may provide a significant resource for molecular testing and may even be the primary option as material for NGS assay.[18] There are multiple advantages reported with molecular testing on supernatant for molecular pathology including (a) high quality of DNA due to the lack of interferences related to the formalin fixation, (b) decreased cost with savings related to handling and processing of FFPE of cell-blocks, (c) faster turnaround time by elimination of handling and processing of FFPE of cell-blocks, and (d) overcome the dependence on the cell-block material which many labs may not be successful in providing dependably. Although it may not be suitable for routine workflow and archival practices of individual cytopathology labs, unstained cytology smears are an excellent source of nucleic acid as an alternative to FFPE cell-blocks.[8]
ii. Processing of cell-blocks for the highest yield of diagnostic tumor cells
Most of the data available in the published literature on ancillary tests, including IHC and molecular pathology tests, are based on the results obtained on FFPE of surgical pathology tissue. Because of this and other additional benefits of providing morphological clues comparable to the traditional tissue sections with the advantages of applying the workflows and other protocols such as archival conditions, FFPE of cell-blocks is preferred for most of the ancillary studies including IHC, FISH, CISH, and molecular pathology testing.
The critical significance of quantitatively and qualitatively optimum FFPE of cell-blocks is an obvious priority. However, this important component has significant variation due to wide institutional differences with a lack of quantitative and qualitative reproducibility.[1,2,17] Almost all of these limitations and challenges are addressed if FFPE of cell-blocks are prepared by NextGen CelBloking™ kits,[26] which would substantially improve the cell-block sufficiency for molecular tests. Another simple but underestimated modification is the proper utilization of scant resources of diagnostic material in the cell-block by avoiding application for the ancillary tests in cases where molecular pathology testing is anticipated (or when the tissue procurement is primary performed for molecular pathology testing). This may be further emphasized in the requisition form provided with the cytology specimen by communicating it to the cytopathologist/pathologist to preserve the cell-block material for elective molecular pathology testing.[27]
iii. Organize molecular laboratory for cytology specimens in the algorithm
Deparaffinization of FFPE of cell-block material is the most vulnerable step with the highest potential to lose diagnostic material especially in cell-blocks and small biopsy specimens. The singly scattered cells and microtissue fragments in the cell-block may be lost during various deparaffinization steps such as pipetting off and processing with various reagent mixtures and ethanol washings.
Mineral oil extraction
Nucleic acid recovery with maximum outcome depends on the proteinase K–based lysis step. Recently, this lysis step is performed by incubating FFPE of the cell-block specimen with mineral oil and lysis solution. Sample purification steps are performed after complete tissue lysis. Mineral oil has been used in molecular testing to prevent evaporation loss with the additional benefit of preventing carryover related loss of diagnostic components during the deparaffinization process. DNA yields from FFPE of cell-blocks are significantly higher after the application of mineral oil for deparaffinization. The DNA yield is roughly doubled as compared to the routine method without mineral oil protocol.[18] Mineral oil extractions also reduce the hands-on labor with a decrease in transfers, centrifugation, and supernatant discarding steps further minimizing the loss of diagnostic material.[28,29]
There are many different commercially available kits for DNA isolation from FFPE samples. Our molecular pathology lab uses Tween20 and heat for deparaffinization for example.
iv. Update bioinformatics services
Large files of NGS generated data require multiple bioinformatic processes prior to its visualization and interpretation of sequence alterations, copy number changes, and structural rearrangements.[19-22] Comparative evaluation of normal tissue is used for canceling out the artifacts related to the sequencing techniques for identifying alterations in copy number. Cell-block material is generally sufficient for evaluating standard mutation analysis; however, identification of copy number alterations requires revalidation and normalization with non-neoplastic tissue sample processed using a similar protocol with efficient copy number evaluation due to the possibility of excess noise, which may interfere with such assessment.
Although having a normal comparator does make identification of tumor-specific changes easier, it is not required. If enough samples are analyzed during the validation process to establish the “average depth of coverage” across targeted regions, then the patient samples can be compared to this platform-specific average to identify copy number changes.
The bioinformatics infrastructure will vary widely based on the type of testing and the platforms used.
In summary, molecular studies can be performed on cell-blocks for sequencing and detecting a variety of “druggable” genetic alterations that have therapeutic benefits [Table 1]. Similarly, molecular testing may be performed on cell-block to determine the site of origin for metastases with some limitations related to the reliability of such results in up to 25% cases,[17] in some rare cases where relatively less expensive, easily available IHC could not be effective in identifying the primary.
With proper organization of different interdisciplinary components of molecular pathology testing on cell-blocks, the success rates of various molecular tests on lung cytology have been reported to be approximately 90% and comparable to that with core biopsies.[18] The cytologic material should be preferred over the core biopsies because of the superior quality of nucleic acid. This leads to a better outcome without the requirement for enriching the tumor cells with technical interventions such as challenging manual macrodissection or microdissection, especially with the tiny diagnostic components in the cell-block. It is important to know the various testing requirements and methodology details to assess the molecular pathology tests. Standardizing and defining specific protocols for handling and processing of cytopathology specimens at the institutional level as an interdisciplinary effort is critical for the best outcome in molecular testing. Because the entire process is interdependent, even the slightest change in protocol could compromise the outcome and performance of the molecular pathology assays. Any changes, including simple components such as communication, require seamless interaction between the clinical, cytopathology, molecular, and bioinformatics divisions. The new or revised protocol should be validated and executed only after evaluating the performance.
THE REQUIREMENTS FOR SAMPLE PROCESSING
The requirements for sample processing often is dependent on the testing lab.[30] Many of which require a certain number of slides or tumor content. Other labs may have their own proprietary sample collection kit. For example, the FoundationOne DDx assay requires 40–50 microns of a tumor or a minimum of 25 mm2 sample (10 unstained slides cut at 4-5 microns thick to achieve a tissue volume of 1 mm3). Usually, molecular studies on cell-blocks are performed on the curls of thick paraffin sections. For the cell-blocks prepared by NextGen CelBloking™ kits, the wells with concentrated diagnostic tumor cells can be cored out for molecular pathology testing.[26]
Considerations while selecting tissues for molecular testing [30,31]
The successful molecular and cytogenetic testing is largely dictated by the qualitative integrity and quantitative adequacy of nucleic acid in the specimen under testing.
A single cell typically contains 6 pg (picogram) of DNA and 10–30 pg of RNA. Microarrays, either for RNA or DNA typically require at least 500 ng (nanogram) DNA or RNA. Thus, DNA microarray would require almost 84,000 cells and RNA microarray would need 20,000–50,000 cells. Cell-blocks prepared using enhanced methods such as NextGen CelBloking kits can have a sufficient number of cells. Ongoing research in this area should allow the application of cell-blocks in this area with multiple benefits including the availability of testing with minimally invasive methods.
Transcriptome analysis with NGS can be done with as little as 10 ng of RNA and would be a more appropriate technique for genome-wide RNA expression studies or to detect fusion genes. Targeted assays such as allele-specific PCR and digital droplet PCR are recommended for mutation-based testing.
In contrast to the robustness of DNA molecules and microRNA, messenger RNAs are vulnerable to be destroyed easily by ubiquitous RNase enzyme. Due to this, the collection of specimens for mRNA-based tests should use the methodology to preserve mRNA by inhibiting RNase. A variety of commercial RNase inhibitors including RNAlater® (Invitrogen) are available. Other RNase inhibitors include Guanidinium thiocyanate and formaldehyde (even 1% formaldehyde inhibits RNase).
Guanidinium thiocyanate is a chaotropic agent and degrades protein without preserving tissue morphology. In contrast, 10% formalin (3.7% formaldehyde) is a routine fixative in diagnostic pathology with excellent preservation of tissue morphology. Because of this, many studies have reported and standardized protocols for studying mRNA in FFPE, (although formaldehyde introduces some limitations due to cross-linking issues).
Qualitative tissue considerations [32]
The factors to be considered include the method of tissue collection and processing, the fixative used, the total absolute amount of tumor in the specimen, and the percent tumor nuclei.
Variety of specimens including cell-blocks can be used for molecular testing. FFPE of cell-blocks from fixed tissue collected with a relatively simple, easy, and minimally invasive approach is an important source of nuclei acid for molecular testing. Fixation and processing protocols have to be considered while selecting one of the multiple ways to get nucleic acid. This may in the form of coring out the areas of FFPE of cell-blocks with the highest number and proportion of diagnostic cells. This is facilitated in a reproducible manner in cell-blocks prepared with NextGen CelBloking kits. Another approach is to scrape material from the unstained direct cytology smears.
As compared to formalin, decalcification leads to more damage to the nucleic acids. As a result, such specimens may be inadequate for NGS-based testing. In addition, the availability of a wide spectrum of various decalcification reagents adds to the ambiguity.
The specimen under consideration may potentially have inhibitors and competitive contaminants interfering with the PCR reaction. Due to this, some specimens even with the optimum quantity of tumor cells may not produce desirable results. Some examples of such native inhibitors in tissues include melanin and calcium. Melanin can be easily detected and identified in H&E stained sections, unstained sections, and FFPE blocks. But even with highly melanotic specimens, it is not predictable to anticipate which cases will fail. Because of this, it is worth attempting the molecular test even in specimens with high melanin.
Tissue artifacts such as crush artifact may not allow estimation of tumor percent. Compromised morphology will interfere with ISH assays due to the inability to discern the individual nuclei for counting the signals. For this reason, usually, the tissues with crush-artifact are inadequate for testing. Although not directly related to most of the cytology specimens, cautery artifacts would also be interfering with the scoring of ISH signals. Besides, DNA obtained from such tissue with cautery artifact is inadequate and may not amplify properly.
Similarly, tissue necrosis may interfere with molecular testing. Although tiny foci of necrosis may not interfere with the molecular tests, the specimens with extensive tissue necrosis are inadequate due to suboptimal quality and quantity of nucleic acid.
Quantitative tissue requirements [32]
Major requirements include:
The absolute amount of diagnostic component such as tumor cells
The relative proportion of tumor cells as the percent number of tumor nuclei.
These requirements vary for each test depending on its performance metrics. For example, Lung Cancer-Targeted Gene Panel with Rearrangements at Mayo Laboratories requires 30 ng of DNA, obtainable from approximately 5000 cells from cytology specimens with at least 20% tumor nuclei.[32]
Tests based on FISH do not require DNA extraction, and so even a smaller proportion of intact tumor cells are acceptable for FISH. For NGS-based assays, there are significantly wide requirements depending on the method of testing.
MOLECULAR TEST CATEGORIZATION BASED ON TECHNICAL METHODOLOGY [TABLE 2]
Non-morphology based random molecular tests [33]
Targeted mutation analysis for “hotspot mutations” or common fusion genes:
ARMS-PCR
Real-time PCR using hydrolysis probes or LightCycler chemistry
Digital droplet PCR
RT-PCR for fusion genes.
Expression analysis:
qRT-PCR
Expression microarrays.
Copy number variation:
Oligo arrays – del/dups only
Oligo + SNP arrays - del/dups + loss of heterozygosity.
NGS:
Gene panels, whole exome, or whole genome
RNA seq
Methyl seq.
Method | Types | Remark |
---|---|---|
Random in suspension | PCR: Followed by amplification with PCR • DNA based tests • RNA based tests • Messenger RNA based tests • microRNA based tests |
Starts with DNA or RNA purification |
Morphology based | ISH (FISH-CISH-Radio-labeled ISH): Can be used for DNA, mRNA, miRNA In situ PCR |
Radionucleotide methods are almost extinct. The most predominantly used option is the fluorescence-based method |
PCR | Hot Start PCR Real-time PCR |
|
qPCR and RT-qPCR | a. Droplet digital PCR[40,41] b. Lower density microfluidic chamber based digital PCR |
|
DNA microarrays | a. cDNA microarrays chip b. oligonucleotide/DNA chip |
DNA microarrays permit simultaneous assessment of expression profiling of numerous genes in one assay with the help of two types of DNA microarrays |
Next Gen sequencing[45] | a. Sequencing by hybridization a. Sequencing by synthesis |
Occurs in parallel in very small volumes (nanoliter, picoliter, or zeptoliter) in small spaces at a nominal cost |
Morphology based molecular tests
Targeted chromosomal rearrangements:
In situ hybridization (ISH) (FISH-CISH-Radio-labeled ISH):
Can be applied to DNA, mRNA, miRNA
Radionucleotide methods are almost extinct
Most predominantly used option is the fluorescence-based method.
Testing based on nucleic acid has been a critical diagnostic tool in a variety of clinical settings such as cystic fibrosis, hemochromatosis, infectious processes, and many neoplastic processes. These genetic molecular testing’s are contributing to the ongoing search for specific therapeutic targets, finding gene therapy or biologic response modifiers, assessing disease prognosis with therapy response, detecting minimal residual disease, and many more potential applications in the future.[33]
Genetic molecular tests may be broadly divided into DNA or RNA (messenger RNA or micro RNA) based.
Tests such as copy number analysis, expression analysis, and targeted SNP genotyping are evaluated with DNA microarrays (also called DNA chip, biochip, or DNA chip technology). However, usually, the initial DNA or RNA target has to be amplified with polymerase chain reaction (PCR).
For RNA, a complementary nucleic acid sequence (cDNA) is generated with RT from a retrovirus. This cDNA is then amplified with PCR. This is called RT PCR. The amplified cDNA is then tested or sequenced.
PCR [Figure 2]
PCR is a simple, widely applied molecular biology technique for amplifying DNA and RNA before the application of detection techniques. This initial rapid step is to amplify the scant target nucleic acid for increasing the sensitivity of detection techniques to be applied at later stages.
Because of this, PCR is the backbone for many modern molecular pathology tests. In principle, the oligonucleotide primers flank the area of interest, for producing many copies of the targeted DNA sequence with the novel heat-resistant DNA polymerases (DNA copying enzymes) [Figure 2].
PCR cycle includes three elementary steps in the order mentioned below [Figure 2]
Denaturing (separation of two DNA strands of double-stranded DNA [dsDNA] by heating at 90–95°C)
Annealing or hybridization (binding of oligonucleotide primers with the complementary bases of the single-stranded DNA at 55–68°C depending on the melting temperature of the oligonucleotides)
Polymerization (elongation) by recruiting nucleotides in the sequence of the original cDNA with DNA polymerase at 72°C [Figure 2].
This results in two new DNA strands, one original strand, and the other newly polymerized complementary strand. The cycle is repeated 20–40 times (in 29 cycles, the initial strand would be amplified to get X230 strands) for subsequent testing. PCR may also be applied for amplification of the targets in situ in sections or cytology preparations.[34]
The key ingredients for executing PCR include the DNA template to be amplified, proper primers, thermostable DNA polymerase (which can withstand temperatures over 94°C), nucleotides, magnesium, and a thermocycler (which is an equipment for rapid heating and cooling in a controlled repetitive manner).
Guanine/Cytosine-Rich (GC-Rich) Regions
There are multiple technical issues to be considered while performing this otherwise relatively simple technology. One such consideration is the relative stability of GC-rich regions. Besides, these GC-rich areas tend to form secondary structures, such as hairpin loops. Due to this, such GC-rich areas would be resistant to complete separation during the denaturation phase compromising the next steps. Adjustments are required to overcome these challenges by raising the denaturation temperature to improve denaturation and separation of such two strands in combination with a higher annealing temperature for a shorter duration to avoid non-specific binding of GC-rich primers. Furthermore, the application of some reagents such as DMSO, glycerol, and betaine would assist by disrupting the secondary structures related to GC interactions to facilitate denaturation and separation of the double-stranded nucleic acid.
RT-PCR
RNA as single-stranded nucleic acids is highly unstable and so challenging to work with.
The target RNA sequence is reverse transcribed with RT (an enzyme from retrovirus) to create a complementary strand of DNA (cDNA). The results depend on the purity and quality of the sample RNA template. The initial step in RT-PCR is the creation of a DNA/RNA hybrid. However, RT enzyme also acts as Ribonuclease H, which is a sequence-independent endonuclease enzyme that can cleave RNA in the RNA/DNA substrate through a hydrolytic mechanism. The cDNA strand is then amplified further in the same manner as mentioned above by PCR.
The final amplified material can be analyzed by various techniques including evaluation with fluorescence (fluorescent dye-labeled oligonucleotide primers) such as, analysis with DNA microarrays (DNA chips) or NextGen sequencing (NGS), etc.
Some variations in the basic principle of PCR are described below
Hot start PCR
Routine PCR may lead to non-specific amplification. During PCR, DNA polymerases perform most optimally at 68–72°C, because of which the temperature selected for polymerization is in this range. However, this enzyme may retain DNA polymerase activity even at a lower temperature in the range applied during the annealing phase. As the primers bind non-specifically, it may cause random amplification even after setting up the reaction on ice. A simple approach is the inactivation of DNA polymerase until the PCR starts. This polymerase inhibitor can disengage from the DNA polymerase only at a specific temperature.
The inhibitors could be antibodies or aptamers (chemical antibodies) or affibodies (small, strong proteins which bind to many proteins and peptides similar to monoclonal antibodies) or chemical variations which bind DNA polymerase but denatures at high denaturation temperature.
Real-time PCR/Quantitative PCR (qPCR and RT-qPCR)
“Real-time” PCR allows quantitation of PCR product in real-time. The automated equipment monitors and quantitates PCR product after each cycle in the form of the intensity of fluorescence proportional to the amplification after each PCR cycle. Depending on the number of copies of target DNA in the test sample, the critical copy number may be reached by the 16th or 36th cycles. The real-time PCR is rapid and allows quantitation. Because both amplification and quantitation of the sample are performed in the same sealed container, the chances of contamination are minimized. Multiple samples may be tested in 96 well plates as a single run with the availability of results as early as 2 hours depending upon the instrument used.
Dye-based qPCR
Typically a green fluorescent dsDNA binding dye permits quantification of the amplified DNA. The fluorescence increases as DNA strands are amplified with each cycle permitting quantification in “real-time.” However, this approach can be applied to only one target at a time, because the fluorescent dye will bind to all dsDNA.
Probe-based qPCR
Another approach is probe-based qPCR by which multiple targets can be studied synchronously in the same sample. This technique demands proper standardization and designing of the target-specific probe(s) with primers. Various types of probes are available. The most common is a hydrolysis probe incorporating a fluorophore and quencher. The quencher prevents fluorescence of the fluorophore and nullifies the fluorescence resonance energy transfer (FRET) through the quencher till the probe is complete. The probe is hydrolyzed as the PCR reaction proceeds with primer extension leading to amplification of a particular target. The hydrolysis of the probe breaks the fluorophore from the quencher which translates into the enhancement of fluorescence directly proportional to the extent of the amplification of the target sequence. This methodology is usually preferred for diagnostic assays because it is more specific than random dye-based qPCR. It is also preferred as the release of one fluorophore is equivalent to the amplification of one PCR product.
LightCycler technology
Mechanism: Emission of long-wavelength fluorescence based on the principle of FRET when two fluorophores come in close contact with each other at the probe hybridization temperature.
In this technology, rapid-cycle PCR is combined with real-time monitoring with fluorescence and melting curve analysis. PCR in a closed-tube system with rapid amplification allows product accumulation in 15–30 minutes. Genotyping and haplotyping are possible with melting curve analysis. Mutations could be identified by analyzing melting temperature or curve shape changes.[35]
This generates typical melting curve patterns related to various homozygous and heterozygous states. There are many ongoing advances including dual hybridization probes, multiplex genotyping, and higher multiplexing with novel designs. Recent systems have high versatility with high throughput (96- or 384-well plates) allowing many gene detection protocols, gene expression analysis including variation analysis, array data validation, and many more.[36]
Melt curve analysis can also be performed with the dye-based qPCR. The advantage of the hydrolysis probe PCR is that multiple targets can be quantitated simultaneously. Hydrolysis probe PCR may also be used for genotyping.
In situ PCR
PCR can also be applied at the cellular level in tissue sections and smears for detection of nucleic acid in low concentration in frozen or paraffin-embedded cells or tissue sections of FFPE. It may be targeted for detection and localization of DNA or mRNA (and recently miRNA) in cell organelles, intact cells, or tissue sections as FISH or CISH.[34,37-39]
The steps for in situ PCR include: (a) Tissue preparation, (b) perform PCR (or in situ RT-PCR) on section, and (c) signal visualization (florescent or chromogenic). The process takes about 6 hours. In situ PCR allows the identification of individual cells or tissue to enable the localization of the target nucleic acid under scrutiny.
Fluorescent and Chromogenic in situ hybridization (FISH and CISH)
Oligonucleotide probe (which may be Painting probe, Centromeric probe, or locus-specific probe) labeled with a fluorescent dye (such as Texas red, FITCI green, acridine orange) attach to the cDNA as the target in the cells of tissue sections. The cells with the hybridized target can be easily detected with a fluorescence microscope.
CISH modifies the FISH by replacing the fluorescent dye with a chromogenic signal detection system similar to that used for IHC. CISH is a better option for diagnostic laboratories because of the routine availability of bright-field microscopes. Another advantage of CISH is that the slides can be archived.
Droplet digital PCR (ddPCR) and lower density microfluidic chamber based digital PCR (cdPCR)
ddPCR and cdPCR are a recently available commercial technologies for accurate nucleic acid quantification in a sample. It estimates absolute quantities of nucleic acid molecules encapsulated in discrete, water-in-oil droplets of defined volume. This technology should be suitable for multiple applications, including direct quantification of mRNA to study gene expression, detection of rare alleles, estimation of germline and somatic copy number, and pathogen quantification.[39-41]
DNA MICROARRAYS
DNA microarrays permit simultaneous assessment of expression profiling of numerous genes in one assay with the help of two types of DNA microarrays:
cDNA microarrays chip
Oligonucleotide/DNA chip.
cDNA microarrays chips: Made of numerous DNA microarrays. cDNA sequences complementary to mRNA related to respective genes are immobilized on a single glass slide. The extracted mRNA in the sample specifically hybridize with the immobilized cDNA on the chip. Any mRNA hybridizing with the corresponding cDNA on the chip are tagged with a fluorescent dye (blue, green, yellow, orange, or red). The attachment with the number of fluorescent dye molecules will be proportionate to the number of hybridized mRNA and so the intensity of fluorescence could quantitate the gene expression. The intensity of fluorescence for each gene location represented by respective cDNA is estimated by a microarray reader as a colored dot for each gene spot arranged as a grid in the chip. The results of the microarray reader are compared with the computerized database on the information about various genes in a different location on the grid to identify and estimate the level of gene expression of a particular gene
Oligonucleotide/DNA chips: These silicon chips are similar to cDNA microarrays with the difference that the immobilized DNAs are short length oligos no longer than 25 base instead of cDNA. Because the oligos are shorter, many of these could be accommodated with higher density and allow an increased number of oligos on each chip. However, the problem of non-specific binding because of the high density of oligonucleotide has to be addressed with various approaches built in the chips and by analytic methodologies. This allows testing of more than 12,000 genes on the same chip.
The applications of these chips include analysis and comparison of hundreds of tumors for generating various “fingerprints” of gene expression patterns correlating with a range of prognosis and therapy response for personalized medicine[42,43] including the study of gene expression for identifying the primary site of origin [Table 1].[44] For evaluating tumors of unknown primary origin, chips require only 10–12 critically significant genes for achieving 80%– 88% accuracy.[44] Simple chips like this may be relatively easy to operate in routine diagnostic labs.
Oligonucleotide chips are used for copy number analysis (deletion or duplication analysis) for a subset of genes or the whole genome. Resolution is dependent on probe spacing but is typically 20 kb (kilobases) bases for whole-genome oligonucleotide arrays. If the array includes bi-allelic SNP detection, loss of heterozygosity may also be detected.
NGS[45] [Figures 6 and 7]
The designation as “next-generation” implies that a next “next-next” generation is expected soon as a new technology. The preferred approach should have been to call these as second-generation, third-generation, and so on (abbreviated as G2.0 [G two point O], G3.0 [G three-point O], and so on). However, currently addressed NGS is referred to as a high-throughput technology that allows fast sequencing of DNA and RNA samples including FFPE [Figure 6]. The technology has a wide range of applications which include counting of chromosomes, profiling of gene expression, and checking for epigenetic changes with other molecular testing for various evaluations to enhance patient care with personalized medicine [Table 1 and Figure 3].

- The advantages and limitations of next generation sequencing.

- Next-generation sequencing.
NGS methods broadly fall into two categories:
Semi-conductor sequencing and
Sequencing by synthesis
These new methods occur in parallel in very small volumes (nanoliter, picoliter, or zeptoliter) in small spaces at a nominal cost with ongoing refinements including continued miniaturization leading to a further drop in the cost per base. Furthermore, other approaches including designing large turnover core facilities and planning sequencing centers that deal with pooled larger quantities for discounted prices with saving in labor and other overhead expenses would decrease the cost further.
Future outlook in molecular pathology testing
DNA sequencing is rapidly advancing technology with the final goal of faster and precise results with minimal DNA or RNA input at the lowest cost. The ability to sequence from a single cell or scant circulating nucleic acids is now already clinically available.[45] On the instrumentation side, they would be getting smaller with minimal energy demand including chargeable tiny solar batteries with the requirement of a minimal quantity of reagents with minimum to no maintenance (maybe disposable single-use low-cost kits/devices) is feasible with the ongoing application of nanotechnology, microfluidics, bioinformatics, artificial intelligence, robotics, and other technologies.
PROTEOMICS [FIGURE 3E]
Proteomics is a powerful tool allowing evaluation of gene manifestations directly to the level of protein expression on a large-scale.[46] Technical advances for analyzing particular cells, tissues, or organisms regarding proteins with their functional and structural details in addition to their interactions available as an organized database are rapidly progressing. With continued advances in the instrumentation and methodologies, proteomic analysis is continuously evolving at a rapid pace. The capability to recover protein molecules from FFPE including cell-blocks with targeted or shotgun proteomic analysis has extended many novel prospects in various areas including its application on cell-blocks.
Detection of various proteins as antigens in tissues has been routinely evaluated by IHC in multiple clinical scenarios including evaluation of various cancers. However, this approach is expensive with its application for only a limited number of individual protein epitopes at a time allowing limited quantitative application with challenges related to antibody standardization. On the other hand, mass spectrophotometry (MS) allows quantitative application with highly multiplexed analysis in tissue with precision assays. However, MS depends on proper sample preparation protocols which have been time-consuming in addition to lack of sensitivity for proteins in low concentration.[47]
Another limitation in the past was the dependence on the application of MS on fresh frozen (FF) tissues, which are less practical with the need for expensive archival infrastructure. Because of this, in most clinical situations FF is not a practical option as compared to the relatively well-established alternative with the availability of FFPE which may be stored at room temperature for long periods. Although many nucleic acid-based molecular pathology tests have been well standardized on FFPE,[48] the application of proteomics analysis with MS on FFPE tissues is still evolving.
Recent advances in the application of proteomics have shown comparable results between FF and FFPE tissues.[49,50] Although this is considerable progress, it requires methodical application and standardization for its routine use in proteomics analysis on FFPE tissue in a clinical setting. The areas which need to be addressed include an in-depth understanding of formalin-induced protein alterations addressing potential loss at the protein extraction stage, challenges in quantitative normalization, and reproducibility of MS testing in comparison to the results on IHC.
Most of the proteomics assays with MS are based on proteins extracted from tissue. Matrix-assisted laser desorption/ ionization MS (MALDI-MS) can be used in the mode for imaging which has been mostly performed on FS.[51] However, a few studies have reported its application on FFPE tissue sections.[52,53] For proper progress in this area, it is critical to understand the formalin-protein interaction in tissues during fixation.
Formaldehyde has preferential interaction with primary amines (such as lysine in proteins) and primary amides (asparagine and glutamine) present in tissue for intraand inter-molecular covalent cross-linking of various biomolecules, which may impair the enzymatic activity and also immunoreactivity.[54,55] It had been assumed that due to the covalent nature of cross-linking in formalin-fixed tissue, MS analysis cannot be performed for protein analysis. With increasing experience about antigen retrieval based on heat-induced methods for restoring immunoreactivity, it has also been applied for reversing cross-linking of nucleic acid molecules (RNA and DNA).[56]
Initially reported protein extraction protocol for FFPE tissues for Western blot analysis included 20 min heating at 100°C followed by a 2 h incubation at 60°C in a radioimmunoprecipitation assay pH 7.6 buffer (20 mM TrisHCl, 150 mM NaCl, 1 mM Na2 EDTA 1 mM EGTA 1% NP-40 1% sodium deoxycholate 2.5 mM sodium pyrophosphate 1 mM b-glycerophosphate 1 mM Na3 VO4 1 μg/ml leupeptin) with 2% weight/volume SDS.[57] Later similar protocols have been reported for extraction of proteins from FFPE tissue for shotgun proteomic analysis by different types of MS platforms such as RPLC-MS/MS, SELDI-TOF, and MALDI-TOF/TOF. The basic principle has been the application of heat with surfactant (usually SDS) in an alkaline buffer.[58-63]
However, there are other preanalytical variables, such as duration of ischemia (collection to formalin fixation delay), fixation time, and duration/condition of FFPE blocks which may influence the proteomics results.[63] On another note, tissue processing steps such as dehydration, paraffin-embedding, and duration of FFPE storage do not have a significant effect. A study reported a lack of significant interference due to the storage of up to 10 years on protein profiling.[64]
Comparison of protein profiling with nanoRPLC-MS/MS on 3-year-old FFPE of cell-blocks and fresh cell lysate from lymphoma revealed comparable results for cellular location and molecular function.[62] Even a scant proportion of proteins could be identified in FFPE.
PROTEIN QUANTIFICATION IN FFPE TISSUES
Untargeted shotgun approach
MS has been applied for evaluating proteins in FFPE tissues with an untargeted shotgun approach. The FFPE tissue sample is digested with a proteolytic enzyme to peptides for analysis by LC-MS. However, random digestion introduces significant noise, which may be overcome by applying the technology to specific cells or tissue micro-dissected from FFPE sections. Because of the inability to extract intact protein from FFPE, the approach of analyzing peptide fragments with bidimensional liquid fractionation or immunofixation electrophoresis (IEF) is preferred. This has increased the number of proteins identified with the shotgun approach. However, the expensive and complex processing by this approach requires a significantly longer turn around time. Numerous biomarkers have been reported in oncology and non-oncology areas with the untargeted approach as reported in various reviews.[63,65,66] The availability of high-resolution mass spectrometers has introduced the benefits of analyzing numerous proteins in biological samples, but with the difficulty in analyzing complex results. The recent availability of some biostatistical models would facilitate the analysis of such complex MS data.[67] Most of the evidence support that the results with untargeted proteomic analysis on FFPE tissues are comparable to the well-established targeted techniques such as IHC and other targeted methodologies including selected reaction monitoring (SRM) (mass spectrometry).[68,69]
In summary, shotgun proteomics is a good tool for evaluating various biomarkers. However, it demands complex sample preparation, which hampers its application in routine clinical use.
Targeted shotgun approach
This approach requires previous knowledge about features such as Mass over charge ratio (m/z) of the precursors and fragment ions of the biomarkers to be analyzed. This technique is very specific and highly reproducible with a larger dynamic range and ability to analyze multiple proteins[46] with SRM.[69] Because of the relatively easy availability of FFPE tissue, it would be the preferred sample for analysis with MS in most of the clinical settings. In contrast to IHC, targeted MS analysis is quantitative and permits multiplexing of many analytes on FFPE tissue simultaneously.[46]
CLINICAL APPLICATIONS OF MS ON FFPE TISSUE
Advances in extraction, detection, and quantification for proteomic analysis in FFPE tissue is facilitating applications of MS for studying protein levels and gene expression.[70] MS-based protein analysis offers a quantitative assessment of various tissue proteins simultaneously with SRM as compared to IHC for tumor categorization.[69]
MS-based technology also allows analysis of protein isoforms due to somatic mutations.[71] This is usually difficult with IHC because of the usual lack of availability of antibodies to epitopes specific to a particular isoform.
Analysis and quantification of protein by SRM on FFPE tissue for clinical purposes require the standardization of protocols with proper quality controls with interlaboratory reproducibility. Correlation of results between MS and IHC is a simple approach for the wider application of this evolving technology in a clinical setting.
One of the examples applied currently in clinical setting is combined application of targeted sampling by laser microdissection and analytical power of tandem MS[83] for typing amyloid in FFPE of cell-blocks of anterior fat pad aspirates.[84]
APPLICATION OF PROTEOMICS IN TISSUE SECTIONS [TABLE 3]
Direct scanning of tissue sections by imaging MS (IMS) is now progressing at a rapid pace for application in cancer proteomics. However, clinical material is usually available as FFPE which is regarded most of the time as inappropriate for MS.
Recently a screening method that allows MS analysis for studying multiple tissue samples as tissue microarray (TMA) without extraction or purification of proteins in FFPE is reported as an easy-to-use IMS method.[52] Trypsin treated sections of 2-year-old FFPE of TMA of multiple samples of gastric carcinoma were examined with IMS and detected numerous signals with many differences as compared to the normal tissue. For a summary of protocol to process FFPE sections for IMS, please see Table 3. The study concluded that this method is simple and efficient. It detects and identifies cancer-specific proteins.[52] Similarly, the method can be applied reliably to identifying biomarkers and drug targets. It could also grade the tumors by successfully identifying poorly differentiated areas with high histone H4.
Preparation of FFPE tissue sections 10 μ thick FFPE tissue sections were mounted onto indium-tin-oxide-coated glass slides by lifting the flattened sections floating on warm (50°C) water bath The slides with sections are dried at 45°C The sections are deparaffinized in xylene at 60°C for 10-min and processed by immersing for 5 min each through: twice in 100% ethanol followed by once each in 90% ethanol, 80% ethanol, and 70% ethanol Hydrate the sections and incubate the slides overnight at 55°C in a humid chamber. |
Tryptic digestion Hydrated FFPE sections on glass slides were micro-spotted with trypsin by a chemical inkjet printer After this MALDI target plates with the above glass slides are incubated under high humidity at 37°C overnight |
Matrix deposition A thin layer of 2, 5-dihydroxybenzooic acid (50 mg in 1 mL of 70% methanol/0.1% trifluoroacetic acid) is applied as a matrix on the surface of plates by spraying with 0.2 mm nozzle airbrush The entire tissue surface is covered with a matrix for Co-crystallization with bio-molecules |
Analysis of tissue sections by MALDI mass spectrometry with imaging IMS is performed using orthogonal MALDI The generated MS data are visualized with software such as Bio-Map software (http://www.maldi-msi.org) Molecular images are constructed with this software after baseline correction These mass spectra are aligned with help of SpecAlign software |
Tissue protein identification with statistical analysis The signals showed significantly higher intensity in areas with cancer than in normal tissue |
Acknowledgments
The CytoJournal thanks Erin Wakeling, PhD, FACMGG, Technical Director, DMC Molecular Genetics Laboratory, Detroit Medical Center, Detroit, MI, USA, for conducting an editorial review of this chapter. Author thanks Kathy Rost, Janavi Kolpekwar, and Dr. Moumita Saha Roy Chowdhury for the secretarial and copy-editing support.
Glossary of terminologies
Cell-block: Recommended using instead of other patterns such as “cell block” or “cellblock” to separate “cell block” and “cellblock” related to prison-cell terminologies Cell-blocking: The process of preparing cell block CellBlockistry: The art and chemistry of achieving the capability to handle the tiny components in different types of cytology specimens Cytocrit/Tissuecrit: Proportion of cells/micro-tissue fragments in suspension (comparable to hematocrit) http://alturl.com/87fe5 [Last accessed on 2021 Feb 2]. Needle-rinses: Rinsing of the residual material in FNA needles after preparing direct cytology smears for cytomorphological evaluation.
COMPETING INTERESTS STATEMENT BY ALL AUTHORS
The author’s (VS) spouse has stakes in AV BioInnovation LLC product(s) cited in this review.
LIST OF ABBREVIATIONS (In alphabetic order)
cDNA – Complementary nucleic acid sequence
cdPCR – Lower density microfluidic chamber based digital PCR
CISH – Chromogenic in situ hybridization
ddPCR – Droplet digital PCR
dsDNA – Double-stranded DNA
EUS-FNA – Endoscopic ultrasound guided fine needle aspiration
FF – Fresh frozen
FFPE – Formalin-fixed paraffin-embedded
FISH – Fluorescent in situ hybridization
FNAB – Fine needle aspiration biopsy
FNA – Fine-needle aspiration
FRET – Fluorescence resonance energy transfer
FS – Frozen section
GI – Gastrointestinal
IEF – Immunofixation electrophoresis
IHC – Immunohistochemistry
IMS – Imaging mass spectrophotometry
ISH – In situ hybridization
LCMS – Liquid chromatography followed by MS
LNGPR – Lung cancer-targeted gene panel with rearrangements μ – Microns
MALDI – Matrix-assisted laser desorption/ionization
MALDI-MS – MALDI mass spectrometry
MS – Mass spectrophotometry
nanoRPLC-MS/MS – Nanoflow reverse-phase LCMS/MS
ng – Nanogram
NGS – Next generation sequencing
oMALDI – Orthogonal MALDI
PCR – Polymerase chain reaction
pg – Picogram
qPCR – Quantitative PCR
RPLC – Reverse-phase liquid chromatography
RT – Reverse transcriptase
RT-PCR – Reverse transcriptase PCR
SBS – Sequencing by synthesis
SELDI – Surface-enhanced laser desorption/ionization
SRM – Selected reaction monitoring
TMA – Tissue microarray
TOF – Time of flight
References
- CellBlockistry: Chemistry and art of cell-block making a detailed review of various historical options with recent advances (review) Cytojournal. 2019;16:12.
- [CrossRef] [PubMed] [Google Scholar]
- CellBlockistry: Science of cell-block making as ancillary cytopathology component in the era of minimally invasive techniques with increasing role of molecular pathology (short communication) Clin Surg. 2019;4:2510.
- [Google Scholar]
- Immunocytochemistry of effusion fluids: Introduction to the SCIP approach In: Shidham VB, Atkinson BF, eds. Cytopathologic Diagnosis of Serous Fluids (1st ed). Amsterdam, Netherlands: Elsevier, W B. Saunders Company; 2007. p. :55-78. Ch. 5
- [Google Scholar]
- Cell-Blocks and Hematolymphoid Lesions. Cytojournal 2021 (In print)
- [CrossRef] [PubMed] [Google Scholar]
- Diagnosis of infectious diseases: A cytopathologist's perspective. Clin Microbiol Rev. 1998;11:341-65.
- [CrossRef] [PubMed] [Google Scholar]
- Histological Stains: A Literature Review and Case Study. Glob J Health Sci. 2015;8(3):72-79.
- [CrossRef] [PubMed] [PubMed Central] [Google Scholar]
- Performing and processing FNA of anterior fat pad for amyloid. J Vis Exp. 2010;44:1747.
- [CrossRef] [PubMed] [Google Scholar]
- Molecular Test: NCI Dictionary of Cancer Terms. Available from: https://www.cancer.gov/publications/dictionaries/cancer-terms/def/molecular-test [Last accessed on 2020 Apr 28]
- [Google Scholar]
- Factors affecting the success of next-generation sequencing in cytology specimens. Cancer Cytopathol. 2015;123:659-68.
- [CrossRef] [PubMed] [Google Scholar]
- The feasibility of molecular testing on Cell-blocks created from brush tip washings in the assessment of peripheral lung lesions. J Thorac Dis. 2016;8:2551-5.
- [CrossRef] [PubMed] [Google Scholar]
- Molecular testing guidelines for lung adenocarcinoma: Utility of Cell-blocks and concordance between fine-needle aspiration cytology and histology samples. Cytojournal. 2014;11:12.
- [CrossRef] [PubMed] [Google Scholar]
- Poor Cell-block adequacy rate for molecular testing improved with the addition of DiffQuik-stained smears: Need for better Cell-block processing. Cancer Cytopathol. 2015;123:480-7.
- [CrossRef] [PubMed] [Google Scholar]
- The state of Cell-block variation and satisfaction in the era of molecular diagnostics and personalized medicine. Cytojournal. 2014;11:7.
- [CrossRef] [PubMed] [Google Scholar]
- Laser capture micro-dissection technology. Expert Rev Mol Diagn. 2007;7:647-57.
- [CrossRef] [PubMed] [Google Scholar]
- Role of the surgical pathologist for tissue management in oncology. Bull Cancer. 2013;100:837-45.
- [CrossRef] [PubMed] [Google Scholar]
- Molecular pathology of cancer: How to communicate with disease. ESMO Open. 2016;1:e000085.
- [CrossRef] [PubMed] [Google Scholar]
- Molecular profiling in unknown primary cancer: Accuracy of tissue of origin prediction. Oncologist. 2010;15:500-6.
- [CrossRef] [PubMed] [Google Scholar]
- Optimizing workflows and processing of cytologic samples for comprehensive analysis by next-generation sequencing: Memorial Sloan Kettering cancer center experience. Arch Pathol Lab Med. 2016;140:1200-5.
- [CrossRef] [PubMed] [Google Scholar]
- Poster Presented at, Seattle, WA: 105th Annual Meeting of the United States and Canadian Academy of Pathology.
- [Google Scholar]
- Medical Sciences Animations. Next Generation Sequencing. Available from: https://www.youtube.com/watch?v=shoje_9IYWc [Last accessed on 2020 Apr 24]
- [Google Scholar]
- Egy PharmaScience Competition. Illumina Sequencing Technology. Available from: https://www.youtube.com/watch?v=9YxExTSwgPM [Last accessed on 2020 Apr 24]
- [Google Scholar]
- Next Generation Sequencing (NGS) an Introduction. Available from: https://www.youtube.com/watch?v=jFCD8Q6qSTM&t=464s [Last accessed on 2020 Apr 4]
- [Google Scholar]
- Suitability of thoracic cytology for new therapeutic paradigms in non-small cell lung carcinoma: High accuracy of tumor subtyping and feasibility of EGFR and KRAS molecular testing. J Thorac Oncol. 2011;6:451-8.
- [CrossRef] [PubMed] [Google Scholar]
- Molecular testing guideline for selection of lung cancer patients for EGFR and ALK tyrosine kinase inhibitors: Guideline from the college of American pathologists, International association for the study of lung cancer, and association for molecular pathology. J Thorac Oncol. 2013;8:823-59.
- [CrossRef] [PubMed] [Google Scholar]
- The value of mutational profiling of the cytocentrifugation supernatant fluid from fine-needle aspiration of pancreatic solid mass lesions. Mod Pathol. 2014;27:594-601.
- [CrossRef] [PubMed] [Google Scholar]
- AV BioInnovation, Michigan, USA. Video Resources. Available from: https://www.avbioinnovation.com/resources [Last accessed on 2020 Apr 24]
- [Google Scholar]
- Pathological diagnosis and classification of lung cancer in small biopsies and cytology: Strategic management of tissue for molecular testing. Semin Respir Crit Care Med. 2011;32:22-31.
- [CrossRef] [PubMed] [Google Scholar]
- Deparaffinization with mineral oil: A simple procedure for extraction of high-quality DNA from archival formalin-fixed paraffin-embedded samples. Appl Immunohistochem Mol Morphol. 2014;22:623-6.
- [CrossRef] [PubMed] [Google Scholar]
- High-quality genomic DNA extraction from formalin-fixed and paraffin-embedded samples deparaffinized using mineral oil. Anal Biochem. 2009;395:265-7.
- [CrossRef] [PubMed] [Google Scholar]
- Selecting Pathology Specimens for Solid Tumors Next-Generation Sequencing (Mayo Laboratories Insight) Available from: http://www.alturl.com/i5pxo [Last accessed on 2020 Dec 12]
- [Google Scholar]
- Molecular pathology in anatomic pathology practice. Arch Pathol Lab Med. 2007;132:248-60.
- [CrossRef] [Google Scholar]
- A prospective, multi-institutional diagnostic trial to determine pathologist accuracy in estimation of percentage of malignant cells. Arch Pathol Lab Med. 2013;137:1545-9.
- [CrossRef] [PubMed] [Google Scholar]
- Diagnostic molecular pathology: Current techniques and clinical applications, part I. Proc (Bayl Univ Med Cent). 2003;16:379-83.
- [CrossRef] [PubMed] [Google Scholar]
- High-risk human papillomavirus detection in oropharyngeal, nasopharyngeal, and oral cavity cancers: Comparison of multiple methods. JAMA Otolaryngol Head Neck Surg. 2013;139:1320-7.
- [CrossRef] [PubMed] [Google Scholar]
- LightCycler technology in molecular diagnostics. J Mol Diagn. 2009;11:93-101.
- [CrossRef] [PubMed] [Google Scholar]
- LightCycler® 480 Instrument II (Roche) Available from: http://alturl.com/zw9ha [Last accessed on 2021 eb 02]
- [Google Scholar]
- In situ hybridization detection of microRNAs. Methods Mol Biol. 2010;629:287-94.
- [CrossRef] [PubMed] [Google Scholar]
- miRCURY LNA miRNA ISH Optimization Kits (FFPE) (Quiagen) Available from: http://www.alturl.com/8wr6h [Last accessed on 2020 Dec 12]
- [Google Scholar]
- For Optimization of in situ Hybridizations (ISH) on FFPE Tissue Samples (Qiagen) Available from: http://www.alturl.com/v2r2d [Last accessed on 2020 Dec 12]
- [Google Scholar]
- Evaluation of a droplet digital polymerase chain reaction format for DNA copy number quantification. Anal Chem. 2012;84:1003-11.
- [CrossRef] [PubMed] [Google Scholar]
- Droplet digital PCR as an alternative to FISH for MYCN amplification detection in human neuroblastoma FFPE samples. BMC Cancer. 2019;19:106.
- [CrossRef] [PubMed] [Google Scholar]
- Clinical application of cDNA microarrays in oncology. Oncologist. 2003;8:252-8.
- [CrossRef] [PubMed] [Google Scholar]
- Gene-expression profiles predict survival of patients with lung adenocarcinoma. Nat Med. 2002;8:816-24.
- [CrossRef] [PubMed] [Google Scholar]
- Organ-specific molecular classification of primary lung, colon, and ovarian adenocarcinomas using gene expression profiles. Am J Pathol. 2001;159:1231-8.
- [CrossRef] [Google Scholar]
- Overview of next generation sequencing technologies. Curr Protoc Mol Biol. 2018;122:e59.
- [CrossRef] [PubMed] [Google Scholar]
- Applications of mass spectrometry for quantitative protein analysis in formalin-fixed paraffin-embedded tissues. Proteomics. 2014;14:441-51.
- [CrossRef] [PubMed] [Google Scholar]
- The fundamental flaws of immunoassays and potential solutions using tandem mass spectrometry. J Immunol Methods. 2009;347:3-11.
- [CrossRef] [PubMed] [Google Scholar]
- Measurement of gene expression in archival paraffin-embedded tissues: Development and performance of a 92-gene reverse transcriptase-polymerase chain reaction assay. Am J Pathol. 2004;164:35-42.
- [CrossRef] [Google Scholar]
- Setting proteins free: Progresses and achievements in proteomics of formalinfixed, paraffin-embedded tissues. Proteomics Clin Appl. 2012;6:7-21.
- [CrossRef] [PubMed] [Google Scholar]
- Proteomic studies of formalin-fixed paraffin-embedded tissues. Expert Rev Proteomics. 2013;10:165-77.
- [CrossRef] [PubMed] [Google Scholar]
- MALDI imaging mass spectrometry and analysis of endogenous peptides. Expert Rev Proteomics. 2013;10:381-8.
- [CrossRef] [PubMed] [Google Scholar]
- Imaging mass spectrometry of gastric carcinoma in formalin-fixed paraffin-embedded tissue microarray. Cancer Sci. 2010;101:267-73.
- [CrossRef] [PubMed] [Google Scholar]
- Imaging the clear cell renal cell carcinoma proteome. J Urol. 2013;189:1097-103.
- [CrossRef] [PubMed] [Google Scholar]
- "Tissue surrogates" as a model for archival formalin-fixed paraffin-embedded tissues. Lab Invest. 2007;87:836-46.
- [CrossRef] [PubMed] [Google Scholar]
- DNA extraction from archival formalin-fixed, paraffin-embedded tissue sections based on the antigen retrieval principle: Heating under the influence of pH. J Histochem Cytochem. 2002;50:1005-11.
- [CrossRef] [PubMed] [Google Scholar]
- Extraction and analysis of diagnostically useful proteins from formalin-fixed, paraffin-embedded tissue sections. J Histochem Cytochem. 1998;46:397-403.
- [CrossRef] [PubMed] [Google Scholar]
- Identification of protein-protein interactions using in vivo cross-linking and mass spectrometry. Proteomics. 2004;4:3845-54.
- [CrossRef] [PubMed] [Google Scholar]
- Proteomic analysis of formalin-fixed prostate cancer tissue. Mol Cell Proteomics. 2005;4:1741-53.
- [CrossRef] [PubMed] [Google Scholar]
- Efficient method for the proteomic analysis of fixed and embedded tissues. J Proteome Res. 2005;4:2404-11.
- [CrossRef] [PubMed] [Google Scholar]
- Liquid tissue: Proteomic profiling of formalin-fixed tissues. Biotechniques. 2005;1:32-5.
- [CrossRef] [PubMed] [Google Scholar]
- Identification of proteins from formalin-fixed paraffin-embedded cells by LC-MS/MS. Lab Invest. 2005;85:1405-15.
- [CrossRef] [PubMed] [Google Scholar]
- Analysis of the formalin-fixed paraffin-embedded tissue proteome: Pitfalls, challenges, and future prospectives. Amino Acids. 2013;45:205-18.
- [CrossRef] [PubMed] [Google Scholar]
- Proteomic analysis of formalin-fixed paraffin-embedded renal tissue samples by label-free MS: Assessment of overall technical variability and the impact of block age. Proteomics Clin Appl. 2013;7:273-82.
- [CrossRef] [PubMed] [Google Scholar]
- Toward deciphering proteomes of formalin-fixed paraffin-embedded (FFPE) tissues. Proteomics. 2012;12:1045-58.
- [CrossRef] [PubMed] [Google Scholar]
- Diagnostic Molecular Pathology, Part 2: Proteomics and Clinical Applications of Molecular Diagnostics in Hematopathology, Vol 18. Baylor University Medical Center Proceedings 2005:7-12.
- [CrossRef] [PubMed] [Google Scholar]
- Statistical protein quantification and significance analysis in label-free LC-MS experiments with complex designs. BMC Bioinform. 2012;13:1-17.
- [CrossRef] [PubMed] [Google Scholar]
- Quantitative proteomic analysis of formalin fixed paraffin embedded oral HPV lesions from HIV patients. Open Proteomics J. 2008;1:40-5.
- [CrossRef] [PubMed] [Google Scholar]
- Selected reaction monitoring for quantitative proteomics: A tutorial. Mol Syst Biol. 2008;4:1-14.
- [CrossRef] [PubMed] [Google Scholar]
- New frontiers in proteomics research: A perspective. Int J Pharm. 2005;299:1-18.
- [CrossRef] [PubMed] [Google Scholar]
- Proteoform: A single term describing protein complexity. Nat Methods. 2013;10:186-7.
- [CrossRef] [PubMed] [Google Scholar]
- Prospective gene signature study using microRNA to identify the tissue of origin in patients with carcinoma of unknown primary. Clin Cancer Res. 2011;17:4063-70.
- [CrossRef] [PubMed] [Google Scholar]
- microRNA evaluation of unknown primary lesions in the head and neck. Mol Cancer. 2009;8:127.
- [CrossRef] [PubMed] [Google Scholar]
- Molecular gene expression profiling to predict the tissue of origin and direct site-specific therapy in patients with carcinoma of unknown primary site: A prospective trial of the Sarah cannon research institute. J Clin Oncol. 2013;31:217-23.
- [CrossRef] [PubMed] [Google Scholar]
- CancerTYPE ID®; bioTheranostics Inc, San Diego, CA, USA. Available from: https://www.cancertypeid.com/hcp-the-technology [Last accessed on 2020 Dec 12]
- [Google Scholar]
- Epigenetic profiling to classify cancer of unknown primary: A multicentre, retrospective analysis. Lancet Oncol. 2016;17:1386-95.
- [CrossRef] [Google Scholar]
- Genomic Testing, FoundationOne®, Foundation Medicine, Cambridge, MA, USA. Available from: https://www.foundationmedicine.com/portfolio [Last accessed on 2020 Dec 12]
- [Google Scholar]
- bioT3 Metastatic Cancer Solution. Available from: http://www.alturl.com/nc79y [Last accessed on 2020 Dec 12]
- [Google Scholar]
- Cisplatin in combination with either gemcitabine or irinotecan in carcinomas of unknown primary site: Results of a randomized phase II study--trial for the French study group on carcinomas of unknown primary (GEFCAPI 01) J Clin Oncol. 2003;21:3479-82.
- [CrossRef] [PubMed] [Google Scholar]
- Cisplatin alone or combined with gemcitabine in carcinomas of unknown primary: Results of the randomised GEFCAPI 02 trial. Eur J Cancer. 2012;48:721-7.
- [CrossRef] [PubMed] [Google Scholar]
- MicroRNA stability in postmortem FFPE tissues: Quantitative analysis using autoptic samples from acute myocardial infarction patients. PLoS One. 2015;10:e0129338.
- [CrossRef] [PubMed] [Google Scholar]
- Comprehensive genomic profiling of carcinoma of unknown primary site: New routes to targeted therapies. JAMA Oncol. 2015;1:40-9.
- [CrossRef] [Google Scholar]
- Classification of amyloidosis by laser microdissection and mass spectrometry-based proteomic analysis in clinical biopsy specimens. Blood. 2009;114:4957-9.
- [CrossRef] [PubMed] [Google Scholar]
- Updates in processing of anterior fat pad aspirate for amyloid (with video and sketches) Cytojournal. 2020;17:15.
- [CrossRef] [PubMed] [Google Scholar]